Translate this page into:
Prospects of mesenchymal stem cells in veterinary regenerative medicine and drug development
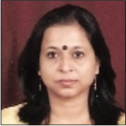
*Corresponding author: G. Taru Sharma, Division of Physiology and Climatology, ICAR-Indian Veterinary Research Institute, Izatnagar - 243 122, Uttar Pradesh, India. gts553@gmail.com
-
Received: ,
Accepted: ,
How to cite this article: Chandra V, Gugjoo MB, Amarpal, Sharma GT. Prospects of mesenchymal stem cells in veterinary regenerative medicine and drug development. J Reprod Healthc Med 2021;2:2.
Abstract
Stem cells are wonder cells that function silently in an individual to grow and/to regenerate. There are various stem cell types; some especially embryonic stem cells (ESCs) favor individual development while more advanced cells like adult stem cells play mostly repair and tissue matrix secretion role. Among various adult stem cell types, mesenchymal stem cells play an important role to maintain tissue homeostasis. These cells are available in almost all the tissue types and exhibit features similar to the ESCs. These cells are immunoevasive, immune modulatory, and/anti-inflammatory, and bear properties of self-renewal (although limited), multiplication, and differentiation. In addition, these cells are able to migrate and home-in to the distant tissues. All these features make these cells potential candidates for therapeutic applications and drug development. There are various studies that have favored their role in therapeutics and drug development, although more studies and further insights are desired to make stem cell therapy a definitive therapeutic option.
Keywords
Drug development
Mesenchymal stem cell
Regenerative medicine
Therapeutics
Veterinary medicine
INTRODUCTION
Stem cells are primitive cells that bear specific characteristic features such as self-renewal, multiplication, and differentiation, among others. Due to the characteristic features, these cells are considered to provide plethora of biological utilities.[1] These cells have wide range of applications right from the basic biological features to the therapeutic applications. These cells may be utilized to understand basic mechanisms such as stem cell genome regulation and cellular behavior including proliferation, differentiation, apoptosis, immortality, senescence and/ aging, and rejuvenation process. Their applications in drug discovery, drug evaluation, genetic engineering, gene therapy, and most importantly in regenerative medicine can be a big boost to the biological sciences, in general, and medical profession/veterinary profession, in particular. Stem cell as a novel therapeutic tool has given hope to many patients suffering from incurable diseases. It has revolutionized the field of regenerative medicine and tissue engineering and is considered to provide a single stop solution to varied ailments, currently lacking any definitive treatment. Stem cell therapeutic evaluation has shown steep rise over the past two decades and has been conducted both at ex vivo and in vivo systems.[2-4]
During developmental cycle of an individual, various types of stem cells arise. At the beginning, fertilization leads to the development of the zygote with a totipotent capability giving rise to whole individual including fetal membranes. From zygote, trophoblast develops that contains external cell mass and inner cell mass. External cell mass gives rise to the fetal membranes, containing mesenchymal stem cells (MSCs) while inner cell mass harbors pluripotent embryonic stem cells that give rise to an individual except fetal membranes. MSCs are naturally present in almost all the adult tissues to take care of routine wear and tear, thus replacing the damaged cells. Furthermore, due to advanced transgenic techniques, somatic cells have been reprogrammed (dedifferentiated) to the pluripotency known as induced pluripotent stem cells (iPSCs) [Figure 1].[2,5,6] Among various stem cell types, adult stem cells especially MSCs make most of the stem cell therapeutics. It is attributed to the availability of numerous tissue sources, easy harvesting procedures, and lack of ethical and teratogenic issues. Furthermore, these cells offer properties of immunomodulation and/ anti-inflammatory, migration, and homing, in addition to those mentioned above.[7] These cells are being evaluated in preclinical research as well as in clinical trials; although no definitive therapeutics has been developed. These cells can offer therapeutic effects either through the differentiation or through paracrine secretions, although latter one is considered as main mechanism involved in the therapeutics. This has made way for the therapeutic applications of MSCs conditioned medium (CM). MSCs CM contains high levels of angiogenic and antiapoptotic factors such as interleukin-6 (IL-6), vascular endothelial growth factor (VEGF), and monocyte chemoattractant protein -1, which inhibits the apoptosis and may promote angiogenesis.[8] MSCs can be isolated from almost all the body tissues such as bone marrow (BM), fat, dental pulp, tendons, synovial membrane, and fetal membranes such as Wharton’s jelly, amnion, and amniotic fluid. Regardless of the source of isolation, MSCs originated from different tissues share comparable multipotency and immunomodulatory characteristics. However, there may be variations in other key features such as immune phenotype, proliferation rate, and commitment to various differentiation pathways.[9] In addition, cultured cells may be heterogeneous group of cells. To isolate MSCs purely, the International Society for Cellular Therapy has put forth criteria of adherence to plastic, surface antigen expression (Sca-1+, CD166+, CD73+, CD90+, CD105+, CD34-, CD45-, CD11, CD14, CD19, CD79, HLA-DR, and CD31), and in vitro multipotent differentiation potential under specific differentiation conditions.[10,11]
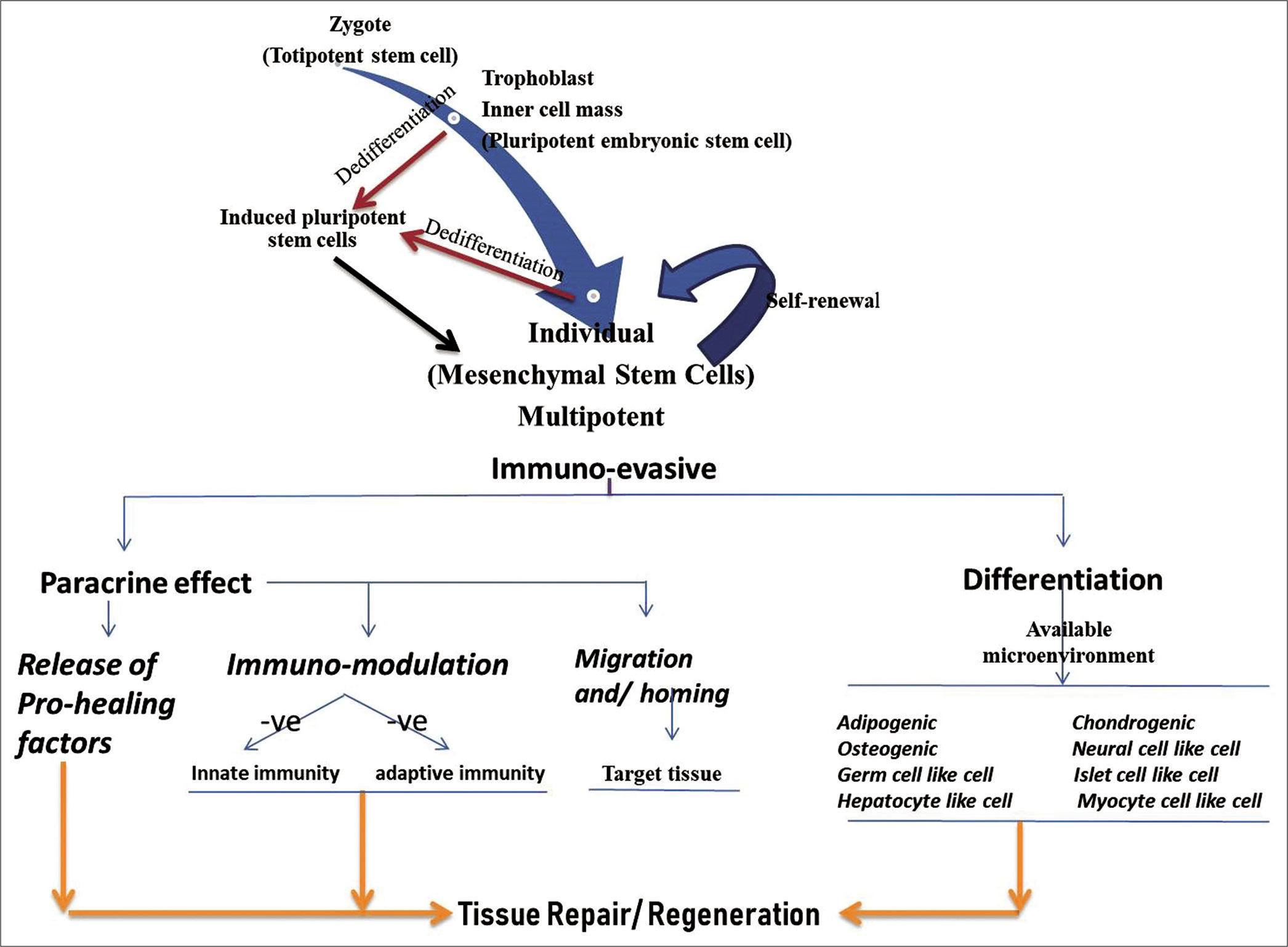
- Stem cells progression at various developmental stages and the possible healing or regenerative role of mesenchymal stem cells.
ROLE OF MSCs IN REGENERATIVE THERAPY
MSCs have potential to repair damaged organs/tissues of the body. Transplanted MSCs can traffic and migrate to injured tissue and consequently home-in to participate in the healing of the tissue. MSCs’ regenerative potential may arise due to their differentiation and trans-differentiation into desired tissue-specific cells, being determined by the local milieu/ microenvironment. This type of repair mechanism, however, remains controversial as the implanted cells remain viable only for limited time and even the differentiated allogeneic or xenogeneic cells may elicit immune reaction.[12] MSCs may secrete chemotactic agents to attract surrounding cells to the particular area to bring about the healing. MSCs on interaction with the resident tissue cells may secrete a wide array of biomolecules including those hampering immune and inflammatory reaction and the ones promoting healing. The MSCs’ CM too has been found to favor wound repair even in diabetic rats.[11,13] Thus, a growing common consensus among the stem cell researchers suggests that therapeutic benefit of MSCs is attributed to the release of biomolecules “paracrine,” rather than a direct cellular contribution.[13,14] Interestingly, microvesicles derived from stem cells have been found to reprogram cells that survived injury and enhance tissue regeneration.[15] Microvesicles, membranous vesicles derived from cells, were previously thought of as artefactual resulting from cell preparatory methods or from cellular debris without any biological purpose. However, there is lot of literature demonstrating their role to transfer genetic information between cells. The microvesicles contain proteins, messenger RNAs (mRNAs), DNAs, and/or microRNAs, which are potentially involved in angiogenic pathways and microRNAs associated cell proliferation, and inhibition of apoptosis at the damages sites.[16]
Immunomodulatory effect of transplanted MSCs
One of the interesting features of MSCs is immune evasive and immune suppression upon transplantation [Figure 1]. A foreign cell engraftment makes adhesion molecules and the major histocompatibility complex (MHC) antigens to interact with the immune cells. MSCs exert an immune tolerant phenotype by expressing low levels of MHC Class I surface antigens and the lack of expression of alloantigens and MHC Class II antigens.[17] The adhesion molecules are constitutively expressed in MSCs, with the exception of ICAM1, being expressed upon induction. MSCs immunomodulatory functions are jointly executed by direct cell-cell contact and the secretory factors.[18] Transplanted MSCs show immunosuppression by modulating the release of soluble anti-inflammatory molecules such as indoleamine 2,3-dioxygenase (IDO) and iNOS pathways.[19] MSCs have been known to secrete a variety of immunosuppressive molecules in humans including programmed death ligand 1, VEGF, IL-10, IL-6, IDO, iNOS, prostaglandin-E2 (PGE 2), hepatocyte growth factor, transforming growth factor β1 (TGF-β1), CXCL-9, CXCL-10, and CXCL-11. However, most of these suppressive factors require a dynamic cross talk between MSCs and T-lymphocytes for their secretion.[20] In addition, Nicola et al. had shown that MSCs are able to inhibit T-lymphocyte proliferation in both mixed lymphocyte culture and in the presence of polyclonal activators (IL-2 or phytohemagglutinin, PHA). CD4 and CD8 T-cells are equally inhibited in dose-dependent manner by MSCs[21] and MSCs-T cells contact leads to T-cell arrest in the G0 phase of cell cycle.[22] MSCs inhibit mitogen-induced T-cell proliferation, as determined by in vitro mixed lymphocyte reactions and transplantation of MSCs across MHC barriers.[23] Other important inhibitory factors secreted by MSCs on interaction with immune effector cells, are PGE-2, IDO, and TGF-β that negatively interfere with T-cell activation and function.[24] IDO converts tryptophan to kynurenine, which leads to T-cell inhibition and activation of immunosuppressive regulatory T-cells (Tregs). MSCs modulate immune responses by the de novo induction and expansion of CD4+ CD25+, FoxP3+, and CD8+ Tregs,[25] which are responsible for inhibiting allogeneic lymphocyte proliferation. The MSC-mediated induction of Tregs is caused not only by direct cell contact between MSCs and CD4+ T cells but also by the secretion of PGE-2 and TGF-β1.[26] MSCs have also potential to block the proliferation of activated B-cells in the presence of INF-ϒ and negatively interfere with antibody production, which depends on dose of MSCs and activation state of the B-cells.[27] MSCs also inhibit chemokine secretion which is responsible for B-cell migration.[28] MSCs inhibit IL-2-induced natural killer (NK) cell proliferation, which is mediated by soluble immunosuppressive factors TGF-β, sHLA-G and PGE-2, and by cell-cell contact.[26] Inhibitory effect of MSCs associated with downregulated expression of the activating NK cell receptors is mediated by PGE-2 and IDO.[29] MSCs also express the toll-like receptors 2, 3, 4, 7, and 9 at the protein level, which affect the immunomodulatory properties of these cells.[30] It is worth mentioning here that the secretome of MSCs may vary based on the tissue source or species involved but a comparable immunomodulatory response may be observed.[3]
MSCs’ POTENTIAL APPLICATIONS IN REGENERATIVE MEDICINE
Wound healing
Cutaneous wound healing is a complex process which requires coordinated cascades of cellular events including inflammation, bio-proliferation, fibroplasia, angiogenesis, and epithelialization.[31,32] In elderly patients, especially diabetics, wound healing is very slow. Delayed wound healing in diabetes is due to diminished migration and proliferation of keratinocytes and fibroblasts, increased cellular apoptosis, inflammatory macrophage phenotype (M1), activation of a pro-inflammatory mediator, reduced vascularization, and impaired recruitment of endogenous progenitor cells toward the injured area.[33] These adverse effects might be due to high glucose microenvironment and elevated levels of inflammatory and immunomodulator cytokines. The anti-inflammatory capacity of MSCs may thus be imperative in the restoration of localized or systemic conditions that are required for normal healing. These patients are more likely to suffer from chronic wounds[34] and thus demand therapeutic options that carries good success rate. MSCs’ application is a new hope by reducing the difficulties to heal such wounds. MSCs improve/circumvent wound healing by stimulating cellular response to injury and promote regeneration rather than scar formation. Xenogeneic canine stem cells and its CM were found to heal experimentally induced diabetic rat wounds at a significantly faster rate of wound contraction and quality of wound healing (rate of epithelization, neovascularization, and collagen deposition).[11] In caprine and equine wound healing models, local implantation of MSCs had led to complete re-epithelialization in shorter period. The healed tissue had shown limited inflammation, thinner granulation tissue, and minimal scar tissue formation.[35-37] Even in equine clinical cases, MSCs had improved wound healing of pressure sores or decubitus ulcers.[38-40]
The current literature demonstrates that MSCs exhibit limited ability to incorporate into the particular tissue and their pro-healing effect is mainly attributed to the trophic mediators being released by them.[41] MSCs could enhance wound healing by creating conducive microenvironment through the release of important biomolecules such as VEGF, insulin-like growth factor, epidermal growth factor, keratinocyte growth factor, stromal cell-derived factor-1, and matrix metalloproteinase-9. MSCs secrete PGE 2 which further regulates inflammation and promotes tissue healing with reduced scar formation. To enhance their delivery, several strategies such as three-dimensional allograft, hydrogel scaffold, and microsphere-based engineered skin loaded with EGF have been found useful.[32] Apart from the stem cell scaffold, various biomolecules such as growth factors, antioxidants, and genetic engineering techniques such as plasmids and other vector-based specific gene transmission may further modulate the stem cells delivery and functionality.
MSCs’ ROLE IN NERVE INJURY/PARALYSIS
Spinal cord injury (SCI) in animals like dog is one of the commonly encountered clinical conditions with resemblance to human condition.[42] Regarding the nature of nerve cells, it is stated that nerve cells have no new cell division after birth in mammals and little is known about its potential to contribute to endogenous repair mechanisms. However, some reports recently evidenced the presence of endogenous stem cell like cells that can differentiate into glial cells, including astrocytes or oligodendrocytes,[43,44] and by which functional neuronal recovery has been reported. Moreover, isolated perivascular MSCs from the human brain could also adopt a glial and neuronal phenotypes, at both mRNA and protein expression. MSCs may act as good in vitro model to study ovine scrapie as the cells express PrPC.[45] MSCs from scrapie affected animal donor had normal trilineage differentiation but carried diminished neurogenic differentiation.[46] This type of model may help to understand disease and develop therapeutics.
Canines and felines are frequently affected with SCI either accidently or mishandling or faulty injections which frequently results in permanent loss of neurological function below injured region. The partial improvement may do occur over a period of time (weeks to months) including both compensatory behavioral strategies to maximize use of spared systems and potential anatomical mechanisms of axonal sprouting[47,48] and remyelination. Injured spinal cord environment could facilitate differentiation along glial lineages. Therefore, it is possible that experimental augmentation of endogenous stem cell responses could increase recovery after injury.[49] As these cells secrete different growth factors which ultimately enhance the recruitment of endogenous stem cells (glia cells) and better results are reported when cases are treated earlier and showed higher recovery rate than delayed treatment. Supplementation of growth factors to MSCs may be promising adjuvant to MSCs to circumvent problems of MSC proliferation and expansion, and survival in-situ.[50]
MSCs in dog and sheep pre-clinical models involving the spinal cord or nerve injuries[51-57] and peripheral nerve injuries[58,59] have been demonstrated to improve outcome. In peripheral nerve injuries, healing although appears delayed but myelinated nerve fibers may regenerate both at distal and the proximal parts of the injury with an overall improvement in nerve action potential.[59] Besides, MSCs and their genetic engineering, many other biological agents such as scaffolds and growth factors have been incorporated. Thus, further refinement in the experimental models is required to compare the role of MSCs and the other biological agents alone or in combination. It is worth mentioning that complete healing and recovery to normal reflexes remain a dream. Thus, further, incites are desired in the field of neurological recovery.
Bone and cartilage repair
Orthopedic problems have been found common among canines and other domestic animals species. Handling of such orthopedic cases has been the cause of concern among canine and feline practitioners worldwide. The therapeutic options, which are clinically available, are currently restricted to allografts, microvascular bone, and osteocytes, myocytes, cutaneously taken either from an autologous donor site, or following bone distraction used for reconstructive purposes. Compared to traditional medicine, stem cell regenerative therapy does not rely on a single target receptor or a single pathway for its action. MSCs improve bone healing through direct differentiation into mature osteoblasts/chondrocytes and/or paracrine effects that facilitate migration and differentiation of resident precursors. MSCs have chondrogenic potential and intraarticular injected MSCs shown chondrogenic differentiation and, in turn, actively produce extracellular matrix.[60] MSCs secrete several biomolecules to make microenvironment favoring stimulation of locally present progenitor cells to repair the damage or by chemoattracting the circulating endogenous progenitor cells to enable repair.[61] MSCs’ paracrine effects can be immunomodulatory, anti-scarring, and chemoattractant. The VEGF is able to activate the formation of a new network of blood capillaries, which is required during the physiological process of bone regeneration.[62] Using biological scaffolds such as PLGA, collagen in combination with MSCs may be most effective strategy for treating bone defects.[63]
Various experimental studies in rabbit and dog have favored the early and much improved bone healing with MSCs with or without the scaffolds and growth factors.[64-66] Even in clinical cases of non-union fracture in dog, application of MSCs, or transplantation of BMP-7 expressing MSC sheets at the fracture site had led to the healing of bone.[67,68] Comparison of MSCs derived from various tissues (adipose tissue, BM, umbilical cord blood, and Wharton’s jelly) had shown comparable improvement in bone healing.[64] In case of cartilage repair, mostly clinical symptoms have subsided with or without actual hyaline cartilage repair.[7,69-74] In cartilage repair, more intensive studies are warranted to yield desired results. In case of bone repair, MSCs appear much promising but further studies are desired to determine the actual posology of the cell therapy.
Tendon/ligament repair
Tendon disorders may arise from the over usage or age-related degeneration, resulting in acute or chronic tendon injuries. Research data suggest that certain tendons are more prone to such insults than others such as the rotator cuff, Achilles, tibialis posterior, and patellar tendons. Tendon and ligament injuries are extremely slow in healing and remain incomplete, posing a major challenge. Application of MSCs at the site of damage proved to be an attractive strategy to improve tendon reparative processes. The use of cultured MSCs for the treatment of tendon injuries is supported by experimental investigations in horses, dog, and laboratory animals where the MSCs were implanted locally and the clinical signs had shown significant improvement including favorable changes in tissue organization, composition, and mechanics of MSC-implanted tendons and ligaments.[1-3] In a clinical study, Renzi et al.[75] reported that the injected BM-MSCs into horses affected by tendonitis or desmitis led to 13 out of the 18 inoculated horses returning to race competitions without any adverse effects. Clinical follow-up by Van Loon et al.[76] reported that 77% of horses (40) returned back to work after the treatment of allogenic MSCs derived from umbilical cord blood. In dog, 9 out of 13 dogs had fully healed cranial cruciate ligament with marked neovascularization and a normal fiber pattern in the areas of injury implanted with MSCs and platelet-rich plasma.[77] In tibial plateau leveling osteotomy repaired cases, adjunct MSCs application had failed to show improved healing as compared to those given NSAIDs.[78] Further, research is required to investigate the exact mechanism of action of MSCs in tendon/ligament repair regarding immunomodulation and trans-differentiation potential.
MSCs IN MAMMARY HEALTH AND MILK PRODUCTION
The mammary gland is a highly specific secretory organ and growth and maintenance of the mammary epithelium depend on the function of mammary stem cells and progenitor cells. Milk production is a function of number and secretary activity of mammary epithelial cells. Transplantation studies in mice have shown that progeny of a single cell could regenerate an entire mammary gland into clear mammary fat pads.[79] Maintaining mammary health and enhancing milk production through stem cell technology are a new and novel concept. The concept is based on the fact that multipotent mammary stem cells (MaSCs) give rise to epithelial precursor cells, the progeny of which develops into either ductal or alveolar cells. An increase in the number of functional alveolar cells will lead to increased milk production. Very less work has been conducted in this area; however, researchers could isolate and characterize MaSCs in from cattle.[80] Appropriate regulation of milk yield, persistency, and dry period, MaSCs can potentially benefit period management and tissue repair. Suitable stimulation of mammary gland stem cells may be helpful for the enhancement of the milk production. BM-derived mesenchymal progenitor cells in conjunction with MaSC are among the most promising vascular progenitors, which can be adopted for therapy of post-mastitis to correct cytological defects. The possibility of increasing milk production by manipulating MaSC is novel. Enhancement of milk production by MaSCs transplantation is based on the hypothesis that expansion of mammary stem cell will produce cascades of proliferation involving progenitor cells followed by differentiated cells. Presence of mammary stem cells in the milk[81] open the possibility to use a noninvasive system for the recovery of primitive cells from the mammary gland that could be a simple and rapid method which can easily provide the necessary amount of cells to monitor the functional status of the bovine mammary gland. The research on bovine stem cells in general and bovine mammary stem cell in particular is very meager and it is hoped that in near future mammary stem cells may prove beneficial in the management of bovine mastitis/ udder health. MSCs in productive mammary gland of cattle and goat may prove double rewarding: On the one hand, MSCs may secrete milk specific proteins in the presence of mammary epithelial or stem cell[82] and, on the other hand, these cells may prevent mastitis by secreting antibacterial proteins.[83] Even in teat fistula or fibrosis MSCs may prove promising by regenerating normal teat tissue.[84,85]
MSCs IN GENETIC ENGINEERING AND DRUG DEVELOPMENT
It has been noted that the response to drugs in animal models may not be the same as in humans. Drug evaluation using in vitro models has been a major boost not only in identifying potential therapeutic compounds but also in increasing our understanding of their absorption, distribution, metabolism, and excretion properties.[86] The use of specialized primary culture models such as hepatocytes, human umbilical endothelial cells, and keratinocytes is limited due to their restricted expandability. Stem cells offer huge potential in the field of pharmaceutical research and regenerative therapy, using medicine. In addition to the obvious health benefits, stem cells may weed out the drugs with dangerous side effects much before they reach the market. This could save the industry millions of dollars in wasted in development costs. Furthermore, since the core competencies are largely driven by academic research, pharmaceutical companies need to gain expertise in the technology. One of the straight forward applications of stem cells lies in clarifying disease mechanisms and toxicity.[87] Testing lead compounds for neuronal, hepatic, and cardiac toxicity would provide direct assessment of the effects and side effects of drugs.[88] MSCs can be used as an alternative to the conventional micronucleus test for screening of genotoxic compounds thus reducing animal uses.[89] Stem cells or iPSCs could also act as transport agents for delivery of small molecules, therapeutic agents, or unmutated normal genes.[90] MSCs tend to grow for long although limited as compared to embryonic/iPSCs but sufficient enough to prove useful in case virus-mediated genetic engineering is a problem. Genetic engineering of MSCs with specific gene may prove useful to help evaluate therapeutic effects besides, its feasibility.
CONCLUSION
Substantial work has been conducted in the past two decades in stem cell research in animal sciences with very promising results, especially for wound and bone healing and nerve injury cases, however, their uses are still in pre-clinical experimental trials. It may be due to the costly affair of stem cell therapy to treat animals even though significant work has been done in canine and equine. Prominent mechanism by which MSCs facilitate regeneration of damaged tissue is by their secretory properties. Stem cell-derived CM has evolved as an alternative therapeutic agent and being cost effective and requiring lesser technical expertise in its application. MSCs possess very promising role in neuroregenerative therapy and the likely mechanisms suggested as the neuroprotection, angiogenesis, axon myelin remodeling, endogenous cell proliferation, and possible replacement of damaged cells. Stem cells also have lot of scope in genetic engineering and drug discovery. MSCs are better alternative for drug discovery and drug testing and this may reduce a lot of laboratory animal sacrifice. Mammary stem cell bears a great hope to enhance milk production but more efforts are needed to explore this opportunity. In animal stem cell therapeutics, though less work has been conducted but there cult to treat various types of diseases and improve productive performances in livestock has very good prospect.
Declaration of patient consent
Patient’s consent not required as there are no patients in this study.
Financial support and sponsorship
Nil.
Conflicts of interest
There are no conflicts of interest.
References
- Mesenchymal stem cell research in veterinary medicine. Curr Stem Cell Res Ther. 2018;13:645-57.
- [CrossRef] [PubMed] [Google Scholar]
- Mesenchymal stem cell research in sheep: Current status and future prospects. Small Rumin Res. 2018;169:46-56.
- [CrossRef] [Google Scholar]
- Equine mesenchymal stem cells: Properties, sources, characterization, and potential therapeutic applications. J Equine Vet Sci. 2019;72:16-27.
- [CrossRef] [PubMed] [Google Scholar]
- Allogeneic mesenchymal stem cells and growth factors in gel scaffold repair osteochondral defect in rabbit. Regen Med. 2020;15:1261-75.
- [CrossRef] [PubMed] [Google Scholar]
- Generation of functional platelets from canine induced pluripotent stem cells. Stem Cells Dev. 2013;22:2026-35.
- [CrossRef] [PubMed] [Google Scholar]
- Derivation of canine induced pluripotent stem cells. Reprod Domest Anim. 2015;50:669-76.
- [CrossRef] [PubMed] [Google Scholar]
- Goat mesenchymal stem cell basic research and potential applications. Small Rumin Res. 2020;183:106045.
- [CrossRef] [Google Scholar]
- Stem cell conditioned media contains important growth factors and improves in vitro buffalo embryo production. Anim Biotechnol. 2016;27:118-25.
- [CrossRef] [PubMed] [Google Scholar]
- Identical, similar or different? Learning about immunomodulatory function of mesenchymal stem cells isolated from various mouse tissues: Bone marrow spleen, thymus and aorta wall. Int Immunol. 2010;22:551-9.
- [CrossRef] [PubMed] [Google Scholar]
- Minimal criteria for defining multipotent mesenchymal stromal cells. The international society for cellular therapy position statement. Cytotherapy. 2006;8:315-7.
- [CrossRef] [PubMed] [Google Scholar]
- Therapeutic potential of canine bone marrow derived mesenchymal stem cells and its conditioned media in diabetic rat wound healing. J Stem Cell Res Ther. 2013;3:141.
- [CrossRef] [Google Scholar]
- Mesenchymal stem cells: Characteristics, sources, and mechanisms of action. Vet Clin North Am Equine Pract. 2011;27:243-61.
- [CrossRef] [PubMed] [Google Scholar]
- Mesenchymal stem cell-conditioned media: A novel alternative of stem cell therapy for quality wound healing. J Cell Physiol. 2020;235:5555-69.
- [CrossRef] [PubMed] [Google Scholar]
- Capillary force seeding of hydrogels for adipose-derived stem cell delivery in wounds. Stem Cells Transl Med. 2014;3:1079-89.
- [CrossRef] [PubMed] [Google Scholar]
- Combinatorial human progenitor cell transplantation optimizes islet regeneration through secretion of paracrine factors. Stem Cells Dev. 2012;21:1863-76.
- [CrossRef] [PubMed] [Google Scholar]
- Mesenchymal stem cell-derived microvesicles protect against acute tubular injury. J Am Soc Nephrol. 2009;20:1053-67.
- [CrossRef] [PubMed] [Google Scholar]
- Mesenchymal stem cells inhibit the formation of cytotoxic T lymphocytes, but not activated cytotoxic T lymphocytes or natural killer cells. Transplantation. 2003;76:1208-13.
- [CrossRef] [PubMed] [Google Scholar]
- Immunomodulation by mesenchymal stem cells and clinical experience. J Intern Med. 2007;262:509-25.
- [CrossRef] [PubMed] [Google Scholar]
- Mechanisms of T-cell immunosuppression by mesenchymal stromal cells: What do we know so far? Biomed Res Int. 2014;2014:216806.
- [CrossRef] [PubMed] [Google Scholar]
- Immunosuppressive effect of mesenchymal stem cells favors tumor growth in allogeneic animals. Blood. 2003;102:3837-44.
- [CrossRef] [PubMed] [Google Scholar]
- Human bone marrow stromal cells suppress T-lymphocyte proliferation induced by cellular or nonspecific mitogenic stimuli. Blood. 2002;99:3838-43.
- [CrossRef] [PubMed] [Google Scholar]
- Bone marrow mesenchymal stem cells induce division arrest anergy of activated T cells. Blood. 2005;105:2821-7.
- [CrossRef] [PubMed] [Google Scholar]
- Mesenchymal progenitor cells differentiate into an endothelial phenotype, enhance vascular density, and improve heart function in a rat cellular cardiomyoplasty model. Circulation. 2003;108:11253-8.
- [CrossRef] [PubMed] [Google Scholar]
- Mechanisms involved in the therapeutic properties of mesenchymal stem cells. Cytokine Growth Factor Rev. 2009;20:419-27.
- [CrossRef] [PubMed] [Google Scholar]
- Interaction of human mesenchymal stem cells with cells involved in alloantigen-specific immune response favors the differentiation of CD4+ T-cell subsets expressing a regulatory/suppressive phenotype. Haematologica. 2005;90:516-25.
- [Google Scholar]
- Immunomodulatory properties and therapeutic application of mesenchymal stem cells. Clin Exp Immunol. 2011;164:1-8.
- [CrossRef] [PubMed] [Google Scholar]
- Role for interferon-gamma in the immunomodulatory activity of human bone marrow mesenchymal stem cells. Stem Cells. 2006;24:386-98.
- [CrossRef] [PubMed] [Google Scholar]
- Human mesenchymal stem cells modulate B-cell functions. Blood. 2006;107:367-72.
- [CrossRef] [PubMed] [Google Scholar]
- Mesenchymal stem cells inhibit natural killer-cell proliferation, cytotoxicity, and cytokine production: Role of indoleamine 2, 3-dioxygenase and prostaglandin E2. Blood. 2008;111:1327-33.
- [CrossRef] [PubMed] [Google Scholar]
- Modulation of adult mesenchymal stem cells activity by toll-like receptors: Implications on therapeutic potential. Mediators Inflamm. 2010;2010:865601.
- [CrossRef] [PubMed] [Google Scholar]
- Tissue engineering and regenerative repair in wound healing. Ann Biomed Eng. 2014;42:1494-507.
- [CrossRef] [PubMed] [Google Scholar]
- Mesenchymal stem cells and cutaneous wound healing: Novel methods to increase cell delivery and therapeutic efficacy. Stem Cell Res Ther. 2016;7:37.
- [CrossRef] [PubMed] [Google Scholar]
- Challenges in the treatment of chronic wounds. Adv Wound Care (New Rochelle). 2015;4:560-82.
- [CrossRef] [PubMed] [Google Scholar]
- Age decreases endothelial progenitor cell recruitment through decreases in hypoxia-inducible factor 1alpha stabilization during ischemia. Circulation. 2007;116:2818-29.
- [CrossRef] [PubMed] [Google Scholar]
- The use of Wharton's jelly-derived mesenchymal stem cells to accelerate second-intention cutaneous wound healing in goat. Iran J Vet Surg. 2008;3:15-26.
- [Google Scholar]
- Effects of transplanted mesenchymal stem cells isolated from Wharton's jelly of caprine umbilical cord on cutaneous wound healing; histopathological evaluation. Vet Res Commun. 2011;35:211-22.
- [CrossRef] [PubMed] [Google Scholar]
- Allogeneic stem cells alter gene expression and improve healing of distal limb wounds in horses. Stem Cells Transl Med. 2017;7:98-108.
- [CrossRef] [PubMed] [Google Scholar]
- Effects of amniotic fluid mesenchymal stem cells in carboxymethyl cellulose gel on healing of spontaneous pressure sores: Clinical outcome in seven hospitalized neonatal foals. Turk J Biol. 2016;40:484-92.
- [CrossRef] [Google Scholar]
- The effects of equine peripheral blood stem cells on cutaneous wound healing: A clinical evaluation in four horses. Clin Exp Dermatol. 2013;38:280-4.
- [CrossRef] [PubMed] [Google Scholar]
- Effects of mesenchymal stem cells isolated from amniotic fluid and platelet-rich plasma gel on severe decubitus ulcers in a septic neonatal foal. Res Vet Sci. 2012;93:1439-40.
- [CrossRef] [PubMed] [Google Scholar]
- Autologous bone marrow-derived cultured mesenchymal stem cells delivered in a fibrin spray accelerate healing in murine and human cutaneous wounds. Tissue Eng. 2007;13:1299-312.
- [CrossRef] [PubMed] [Google Scholar]
- Pilot study: Bone marrow stem cells as a treatment for dogs with chronic spinal cord injury. Regen Med Res. 2014;2:9.
- [CrossRef] [PubMed] [Google Scholar]
- Proliferation of NG2-positive cells and altered oligodendrocyte numbers in the contused rat spinal cord. J Neurosci. 2001;21:3392-400.
- [CrossRef] [PubMed] [Google Scholar]
- Adult neural progenitor cells provide a permissive guiding substrate for corticospinal axon growth following spinal cord injury. Eur J Neurosci. 2004;20:1695-704.
- [CrossRef] [PubMed] [Google Scholar]
- Characterization of mesenchymal stem cells in sheep naturally infected with scrapie. J Gen Virol. 2015;96:3715-26.
- [CrossRef] [PubMed] [Google Scholar]
- The potential of mesenchymal stem cell in prion research. Zoonoses Public Health. 2015;62:165-78.
- [CrossRef] [PubMed] [Google Scholar]
- Functional recovery after lesions of the nervous system IV Structural correlates of recovery in adult subjects. Recovery of function and collateral sprouting in cat spinal cord. Neurosci Res Program Bull. 1974;12:235-9.
- [Google Scholar]
- The injured spinal cord spontaneously forms a new intraspinal circuit in adult rats. Nat Neurosci. 2004;7:269-77.
- [CrossRef] [PubMed] [Google Scholar]
- Endogenous neurogenesis replaces oligodendrocytes and astrocytes after primate spinal cord injury. J Neurosci. 2006;26:2157-66.
- [CrossRef] [PubMed] [Google Scholar]
- Growth factor regulation of proliferation and survival of multipotential stromal cells. Stem Cell Res Ther. 2010;1:32.
- [CrossRef] [PubMed] [Google Scholar]
- Percutaneous transplantation of human umbilical cord blood-derived multipotent stem cells in a canine model of spinal cord injury. J Neurosurg Spine. 2009;11:749-57.
- [CrossRef] [PubMed] [Google Scholar]
- Comparison of canine umbilical cord blood-derived mesenchymal stem cell transplantation times: Involvement of astrogliosis, inflammation, intracellular actin cytoskeletal pathways, and neurotrophin-3. Cell Transplant. 2011;20:1867-80.
- [CrossRef] [PubMed] [Google Scholar]
- Schwann cell-like remyelination following transplantation of human umbilical cord blood (hUCB)-derived mesenchymal stem cells in dogs with acute spinal cord injury. J Neurol Sci. 2011;300:86-96.
- [CrossRef] [PubMed] [Google Scholar]
- Functional recovery of spinal cord injury following application of intralesional bone marrow mononuclear cells embedded in polymer scaffold-two year follow-up in a canine. J Stem Cell Res Ther. 2011;1:110.
- [CrossRef] [Google Scholar]
- Impact of local injection of brain-derived neurotrophic factor-expressing mesenchymal stromal cells (MSCs) combined with intravenous MSC delivery in a canine model of chronic spinal cord injury. Cytotherapy. 2016;19:75-87.
- [CrossRef] [PubMed] [Google Scholar]
- Effect of canine mesenchymal stromal cells overexpressing heme oxygenase-1 in spinal cord injury. J Vet Sci. 2017;18:377-86.
- [CrossRef] [PubMed] [Google Scholar]
- Functional recovery after spinal cord injury in dogs treated with a combination of matrigel and neural-induced adipose-derived mesenchymal stem cells. Cytotherapy. 2012;14:584-97.
- [CrossRef] [PubMed] [Google Scholar]
- Use of tissue-engineered nerve grafts consisting of a chitosan/poly (lacticco-glycolic acid)-based scaffold included with bone marrow mesenchymal cells for bridging 50-mm dog sciatic nerve gaps. Tissue Eng Part A. 2010;16:3779-90.
- [CrossRef] [PubMed] [Google Scholar]
- Peripheral nerve regeneration after experimental section in ovine radial and tibial nerves using synthetic nerve grafts, including expanded bone marrow mesenchymal cells: Morphological and neurophysiological results. Injury. 2014;45(Suppl 4):S2-6.
- [CrossRef] [Google Scholar]
- Cartilage repair in a rat model of osteoarthritis through intraarticular transplantation of muscle-derived stem cells expressing bone morphogenetic protein 4 and soluble Flt-1. Arthritis Rheum. 2009;60:1390-405.
- [CrossRef] [PubMed] [Google Scholar]
- Vascular endothelial growth factor: Basic science and clinical progress. Endocr Rev. 2004;25:581-611.
- [CrossRef] [PubMed] [Google Scholar]
- Adipose-derived mesenchymal cells for bone regereneration: State of the art. Biomed Res Int. 2013;2013:416391.
- [CrossRef] [PubMed] [Google Scholar]
- Comparing the osteogenic potential of canine mesenchymal stem cells derived from adipose tissues, bone marrow, umbilical cord blood, and Wharton's jelly for treating bone defects. J Vet Sci. 2012;13:299-310.
- [CrossRef] [PubMed] [Google Scholar]
- Comparison of autogenic and allogenic bone marrow derived mesenchymal stem cells for repair of segmental bone defects in rabbits. Res Vet Sci. 2013;94:743-52.
- [CrossRef] [PubMed] [Google Scholar]
- Escherichia coli-derived recombinant human bone morphogenetic protein-2 combined with bone marrow-derived mesenchymal stromal cells improves bone regeneration in canine segmental ulnar defects. BMC Vet Res. 2016;12:201.
- [CrossRef] [PubMed] [Google Scholar]
- Implantation of canine umbilical cord blood-derived mesenchymal stem cells mixed with beta-tricalcium phosphate enhances osteogenesis in bone defect model dogs. J Vet Sci. 2008;9:387-93.
- [CrossRef] [PubMed] [Google Scholar]
- Use of stem-cell sheets expressing bone morphogenetic protein-7 in the management of a non-union radial fracture in a toy poodle. J Vet Sci. 2017;18:555-8.
- [CrossRef] [PubMed] [Google Scholar]
- Evaluation of an extracellular matrix-derived acellular biphasic scaffold/cell construct in the repair of a large articular high-load-bearing osteochondral defect in a canine model. Chin Med J (Engl). 2011;124:3930-8.
- [Google Scholar]
- Assessment of the effect of intraarticular injection of autologous adipose-derived mesenchymal stem cells in osteoarthritic dogs using a double blinded force platform analysis. BMC Vet Res. 2014;10:143.
- [CrossRef] [PubMed] [Google Scholar]
- Mesenchymal stem cells with IGF-1 and TGF-? 1 in laminin gel for osteochondral defects in rabbits. Biomed Pharmacother. 2017;93:1165-74.
- [CrossRef] [PubMed] [Google Scholar]
- Canine articular cartilage regeneration using mesenchymal stem cells seeded on platelet rich fibrin: Macroscopic and histological assessments. Bone Joint Res. 2017;6:98-107.
- [CrossRef] [PubMed] [Google Scholar]
- Characterization and therapeutic application of canine adipose mesenchymal stem cells to treat elbow osteoarthritis. Can J Vet Res. 2017;81:73-8.
- [Google Scholar]
- A feasibility study on the use of equine chondrogenic induced mesenchymal stem cells as a treatment for natural occurring osteoarthritis in dogs. Stem Cells Int. 2019;2019:4587594.
- [CrossRef] [PubMed] [Google Scholar]
- Autologous bone marrow mesenchymal stromal cells for regeneration of injured equine ligaments and tendons: A clinical report. Res Vet Sci. 2013;95:272-7.
- [CrossRef] [PubMed] [Google Scholar]
- Clinical follow-up of horses treated with allogeneic equine mesenchymal stem cells derived from umbilical cord blood for different tendon and ligament disorders. Vet Q. 2014;34:92-7.
- [CrossRef] [PubMed] [Google Scholar]
- Partial cranial cruciate ligament tears treated with stem cell and platelet-rich plasma combination therapy in 36 dogs: A retrospective study. Front Vet Sci. 2016;3:112.
- [CrossRef] [Google Scholar]
- Evaluation of the effect of a single intra-articular injection of allogeneic neonatal mesenchymal stromal cells compared to oral non-steroidal anti-inflammatory treatment on the postoperative musculoskeletal status and gait of dogs over a 6-month period after tibial plateau leveling osteotomy: A pilot study. Front Vet Sci. 2017;4:83.
- [CrossRef] [PubMed] [Google Scholar]
- Purification and unique properties of mammary epithelial stem cells. Nature. 2006;439:993-7.
- [CrossRef] [PubMed] [Google Scholar]
- Bovine mammary stem cells: New perspective for dairy science. Vet Q. 2014;34:52-8.
- [CrossRef] [PubMed] [Google Scholar]
- Breastmilk is a novel source of stem cells with multilineage differentiation potential. Stem Cells. 2012;30:2164-74.
- [CrossRef] [PubMed] [Google Scholar]
- Co-culture with umbilical cord mesenchymal stem cells promotes the synthesis and mechnism of milk protein in bovine mammary epithelial cells. Xi Bao Yu Fen Zi Mian Yi Xue Za Zhi. 2017;33:185-9.
- [Google Scholar]
- Bovine fetal mesenchymal stem cells exert antiproliferative effect against mastitis causing pathogen Staphylococcus aureus. Vet Res. 2019;50:25.
- [CrossRef] [PubMed] [Google Scholar]
- Mesenchymal stem cell: Basic research and potential applications in cattle and buffalo. J Cell Physiol. 2018;234:8618-35.
- [CrossRef] [PubMed] [Google Scholar]
- Mesenchymal stem cell basic research and applications in dog medicine. J Cell Physiol. 2019;234:16779-811.
- [CrossRef] [Google Scholar]
- Targeting drug transporters-combining in silico and in vitro approaches to predict in vivo. Methods Mol Biol. 2010;637:65-103.
- [CrossRef] [PubMed] [Google Scholar]
- Stem cells in toxicology: Fundamental biology and practical considerations. Toxicol Sci. 2011;120(Suppl 1):S269-89.
- [CrossRef] [PubMed] [Google Scholar]
- Human pluripotent stem cells in drug discovery and predictive toxicology. Biochem Soc Trans. 2010;38:1051-7.
- [CrossRef] [PubMed] [Google Scholar]
- Human mesenchymal stem cells as a novel platform for simultaneous evaluation of cytotoxicity and genotoxicity of pharmaceuticals. Mutagenesis. 2015;3:391-9.
- [CrossRef] [PubMed] [Google Scholar]
- Stem cells as therapeutic vehicles for the treatment of high-grade gliomas. Neuro Oncol. 2012;14:256-65.
- [CrossRef] [PubMed] [Google Scholar]