Translate this page into:
Testicular enzyme activity alterations in rats with liver cirrhosis induced by alcohol and acetaminophen
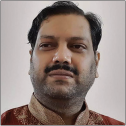
*Corresponding author: Raj Kumar Koiri, Department of Zoology, Dr. Harisingh Gour Vishwavidyalaya, Sagar, Madhya Pradesh, India. rkkoiri@dhsgsu.edu.in
-
Received: ,
Accepted: ,
How to cite this article: Dash D, Thakur P, Koiri RK. Testicular enzyme activity alterations in rats with liver cirrhosis induced by alcohol and acetaminophen. J Reprod Healthc Med. 2024;5:13. doi: 10.25259/JRHM_18_2024
Abstract
Alcohol-induced acetaminophen (APAP) toxicity is one of the numerous factors that might result in liver cirrhosis (LC). The hepatotoxicity of APAP appears to increase in chronic drinkers, according to a number of investigations. These people not only have a greater risk of experiencing acute overdose-related severe and deadly liver damage but are also at risk of experiencing similar substantial liver damage from therapeutic APAP use. The male reproductive system consists of both testes and a few other auxiliary sexual organs. Smaller and lighter testicles are indicative of severe cirrhosis causing testicular atrophy. In the case of severe LC, low testosterone results from hypogonadotropic hypogonadism. Sexual dysfunction is a prevalent condition that is frequently overlooked in individuals suffering from cirrhosis and chronic liver disease. Eighteen rats were randomly divided into three groups. Rats of normal control group received water and normal diet ad libitum; alcohol control and LC group received 4.5% alcohol and a combination of 4.5% alcohol and APAP (300 mg/kg bw) through drinking water, respectively, for 7 days. Several glycolytic and antioxidant enzymes were assessed for their effects through non-denaturing polyacrylamide gel electrophoresis analysis and enzyme activity. The results indicated that long-term alcohol consumption and APAP medication altered the levels of antioxidant and glycolytic enzymes. To the best of our knowledge, this is the first study demonstrating that chronic alcoholism and APAP induce hepatotoxicity, and LC further affects the antioxidant and glycolytic enzyme activities of the testes.
Keywords
Liver cirrhosis
Alcohol
Acetaminophen
Testicular atrophy
Antioxidant pathway
Glycolytic pathway
INTRODUCTION
The two testes, along with a number of auxiliary sexual structures, make up the male reproductive system’s main sex organs. Male sex hormone synthesis and spermatogenesis are two crucial processes carried out by the adult mammalian testis. The organ in question enables the differentiation of diploid postnatal germline stem cells into haploid male gametes. The steroid hormone testosterone, which is also produced by the testis, is in charge of preserving the spermatogenic process as well as secondary male sexual traits. In addition, among its many other functions, testosterone regulates bone density and muscular mass in various organ systems. Making testosterone from its precursor, cholesterol, is a process known as “steroid formation.”[1]
The last common pathological pathway of liver damage resulting from a wide range of chronic liver disorders is liver cirrhosis (LC).[2,3] Alcohol-related liver disease, also known as alcohol-associated liver disease, chronic viral hepatitis B and C, and non-alcoholic fatty liver disease are the most common causes of cirrhosis worldwide.[4,5] The hepatic angioarchitecture is distorted due to histological structural abnormalities in cirrhosis, which increases resistance to portal blood and is the first cause of portal hypertension.[6,7] An initial increase in portal pressure caused by an increase in intrahepatic vascular resistance is the cause of circulating anomalies.[8] The protective barrier that separates the bloodstream from the gut is maintained by a healthy liver,[9] but liver failure and portosystemic shunting in cirrhosis impair this defense system. Reduced hepatic function, immunological dysfunction, and portal hypertension are the hallmarks of decompensated cirrhosis.[10]
Alcohol and acetaminophen (APAP) toxicity is one of the possible causes of cirrhosis of the liver. The hepatotoxicity of APAP appears to increase in chronic drinkers, according to a number of investigations. These people not only have a greater risk of experiencing acute overdose-related severe and deadly liver damage but are also at risk of experiencing similar substantial liver damage from therapeutic APAP use.[11] As expected, long-term alcohol consumption also causes animals to produce microsomal enzymes, which heightens the hepatotoxicity and metabolic activation of APAP. It made sense, under these circumstances, to assume that chronic alcohol consumption may have a comparable potentiation effect on APAP hepatotoxicity after overdosing. Early investigations revealed that individuals with potentially severe APAP intoxication seemed to have a worse prognosis than non-induced patients with similar symptoms.[12]
The previous studies have shown that severe histological abnormalities and smaller, lighter testicles are indicative of advanced cirrhosis in rats. These anomalies included smaller tubular widths, the disappearance of the germinal line, reduced cellular proliferation, spermatogenesis, and a decrease in the expression of testicular transferrin, and a reliable indicator of the integrity of the blood-testis barrier.[13] Oxidative stress increases in the testes as a result of LC, severely impairing testicular function. In men with severe hepatic cirrhosis, hypogonadotropic hypogonadism results in low testosterone,[14] with an additional factor being enhanced peripheral androgen-to-estrogen conversion.[15]
Sexual dysfunction is a prevalent condition that is frequently overlooked in individuals suffering from cirrhosis and chronic liver disease. Patients and healthcare professionals’ reluctance to talk about sexual dysfunction leads to a decrease in the problem’s detection and treatment, which lowers the quality of life.[16,17] A number of conditions are classified as sexual dysfunction, including poor libido, difficulty achieving an orgasm, and erectile dysfunction (ED) in males. The severity of liver illness seems to be correlated with the degree of sexual dysfunction. Almost 55% of individuals with compensated cirrhosis have ED.[18-20] According to a recent meta-analysis, the prevalence of ED increased from 54% in patients with compensated cirrhosis to 88% in those with decompensated cirrhosis, and the severity of ED increased in decompensated patients.[21]
MATERIAL AND METHODS
Chemicals
APAP was procured from Cipla Pvt. Ltd., India. Other chemicals were of analytical grade and purchased from HiMedia Laboratories, Pvt. Ltd., in Mumbai, India.
Experimental animals and treatment
A total of 18 male albino rats weighing 150 ± 25 g were randomly divided into three different groups. Food and water were provided ad libitum. Rats in the normal control group (N) alcohol control (AC) group were given 4.5% alcohol, and the LC group was given a combination of 4.5% alcohol and APAP (300 mg/kg bw) in their drinking water for 1 week. All animal-related procedures were conducted according to the requirements and approval of the Institutional Animal Ethics Committee (No. 379/CPCSEA/IAEC-2021/009).
Preparation of testes homogenate
After the completion of treatment, 3–4 rats from each group were sacrificed by cervical dislocation, and testicular tissues were dissected. Tissues were immediately washed with ice-cold normal saline water, frozen, and stored at −80°C until they were used for biochemical studies. A 10% testes homogenate was prepared by homogenization in ice-chilled 20 mM Tris buffer (pH 7.4), and the supernatant was obtained after centrifugation at 16,000× g for 30 min. The protein concentration was determined using Bovine Serum Albumin (BSA) as a standard.[22]
Measurement of antioxidant enzymatic activity: Superoxide dismutase (SOD), catalase (CAT), glutathione S-transferase (GST), and glutathione (GSH)
SOD
SOD activity was tested in our laboratory[23] using a slightly modified version of the methodology outlined earlier.[24] 1 mL SOD assay cocktail consisted of 12 mM L-methionine, 70 M nitro blue tetrazolium (NBT), 3 M ethylenediaminetetraacetic acid, and 0.1 M potassium phosphate buffer. The cytosolic portions (pH 7.8) of the testis homogenate were added immediately. Twenty microliters of testis supernatant and 30 microliters of 0.2 mM riboflavin were added to the cocktail mixture to initiate the reaction. After that, it was incubated in the presence of daylight at room temperature. As a control, a second set of identical reaction mixture tubes was maintained at ambient temperature and incubated in the dark. At 560 nm, the absorbance of the reaction mixture-containing test tubes and control test tubes with the reaction mixture alone was determined. By comparing the absorbance changes in the light and dark test tubes, the quantity of enzyme required to block NBT breakdown by 50% per min was used to calculate the activity of SOD.
CAT
CAT activity was determined by applying a previously described method.[25] The suitable cytosolic fractions of the testis homogenate were added to a standard 1 mL of assay solution containing 0.5 M sodium potassium phosphate buffer, and the breakdown of hydrogen peroxide (H2O2) at 240 nm was used to measure the CAT activity. A decrease in absorbance at 240 nm was measured every 30 s for 3 min. The results were expressed in units/mg of protein.[26]
GST
The activity of GST was analyzed using, with a minor modification, a previously described methodology.[27] Reduced GSH and 1-chloro-2,4-dinitrobenzene (CDNB) were used as reaction substrates in the GST activity assay. To perform the test, 10 μL of 100 mM GSH, 10 μL of 100 mM CDNB, and 980 μL of PBS (pH 6.5) were combined to create a 1 mL assay cocktail. 10 μL of testes extract was added to the cocktail to start the reaction, and after 5 min, an increase in the optical density (OD) at 340 nm was observed. The results are presented as U/mg of protein. There was a clear correlation between GST activity and the increase in absorbance.
GSH
The total GSH assay was performed on the testes sample in compliance with a previously published methodology.[28] To summarize, 10 μL of testis supernatant was mixed with 1 M Tris-Cl (pH 8.0) and 2 mM dithio-bis (2-nitrobenzoic acid). After 15 min, the reaction mixture was incubated at 37°C. When the absorbance of the supernatant was measured at 412 nm, a yellow color was observed. GSH concentrations were expressed as μM/mg protein.
Measurement of glycolytic enzymatic activity
Lactate dehydrogenase (LDH)
Our laboratory assessed the activity of LDH by slightly altering previously described protocols.[29] 1 mL of the reaction mixture contained 20 mM Tris-Cl (pH 7.4), 6 mM nicotinamide adenine dinucleotide reduced, and 1 mM sodium pyruvate. An appropriate volume of tissue extract was applied to initiate the reaction, and for 5 min, the absorbance at 340 nm was measured every 60 s. The results were expressed in units/mg of protein.[30]
Analysis of SOD, CAT, GST by nondenaturing polyacrylamide gel electrophoresis (PAGE)
SOD
The activity of each antioxidant enzyme was measured using testes extracts and non-denaturing PAGE,[31] with slight modifications, as previously reported.[32] 160 μg of supernatant was added to 12% non-denaturing polyacrylamide gel followed by gel electrophoresis. After electrophoresis, the gels were removed from the chamber and placed in a substrate-specific staining solution for the SOD enzyme in dark for 30 min, followed by light for 10 min. Holding the gel under light caused achromatic bands to appear against a purple backdrop. The staining solution for the SOD enzyme consisted of 0.25 mM NBT, 28 mM riboflavin, and 28 mM tetramethylethylenediamine (TEMED). O2 was produced in the staining mixture as a result of the enzymatic activity of TEMED and the photo-oxidative activity of riboflavin. Reduction of NBT yielded formazan, a purple‒blue substance. Because the active SOD in the gel competes for oxygen and prevents the production of formazan, the gel has an unstained patch against a rich purple background.
CAT
160 μg of supernatant was added to each well of 8% non-denaturing polyacrylamide gel, followed by gel electrophoresis to evaluate the CAT activity. After gel electrophoresis, the gel was submerged in a 0.0003% H2O2 solution for 15 min in the dark. After incubation, the gel was washed twice in triple distilled water (TDW), and the H2O2 solution was removed. Two distinct staining solutions were made: One containing 1% ferric chloride (w/v) and the other containing 1% potassium ferricyanide (w/v). Achromatic bands began to appear on the gel when both solutions were poured onto it. After the development of the achromatic bands, the gel was washed in TDW. The gel densitometry program was used to measure the bands’ intensity. Mean ± standard deviation (SD) was used to express the relative densitometry from three PAGE repeats. The basic principle of CAT staining is that the enzyme in the gel converts H2O2 to water. Without H2O2, potassium ferricyanide cannot be converted to potassium ferrocyanide, which interacts with ferric chloride to produce a blue precipitate. CAT was visible in the gel when yellowish-white achromatic bands appeared on the greenish-blue gel.
GST
To track the GST activity, a 10% polyacrylamide gel was electrophoresed in native PAGE buffer for 3 h at 100 V and 4°C after 160 μg of supernatant was added to each well. After electrophoresis, the gel was allowed to equilibrate in 0.1 M potassium phosphate buffer (pH 6.5) for 10 min. Subsequently, the gel was incubated at room temperature for 10 min using a reaction mixture consisting of 0.1 M potassium phosphate buffer (pH 6.5), 4.5 mM GSH, and 1 mM CDNB and NBT. The gel was incubated for an additional 10 min at room temperature in the dark with 3 mM phenazine methosulfate (PMS) dissolved in 0.1 M Tris-Cl (pH 9.6), after the reaction liquid was discarded. Then, light was applied to the gel, resulting in the appearance of bands indicating activity against a blue background. The densities of the enzyme bands were determined using gel densitometry software. PAGE was conducted 3 times, and the relative densitometric values are shown as the mean ± SD.
Analysis of LDH isozymes
Our laboratory used the protocol of non-denaturing PAGE with a few modest modifications to examine the expression pattern of LDH isozymes.[32] In summary, 160 μg of extracts were added to each non-denaturing 10% PAGE lane, and these extracts were subjected to electrophoresis at 4°C. After electrophoresis, the gels were stained with a particular dye for the substrate using a solution containing 0.125 M Tris-Cl (pH 7.4), 0.5 mM magnesium chloride (MgCl2), 1 mg/mL nicotinamide adenine dinucleotide (NAD), 0.1 mM Li-lactate, 0.25 mg/mL nitro blue tetrazolium, 0.01 M sodium chloride (NaCl), and 0.025 mg/mL PMS. LDH isozymes appeared as dark smears on a white background.
Measurement of lipid peroxidation (LPO)
LPO was measured using a previously described method.[33,34] Utilizing this method, two milligrams of thiobarbituric acid and one milligram of malonaldehyde (MDA) are combined in an acidic solution to produce tri-methionine, a pink substance with a maximum absorbance at 548 nm. After adding the necessary amount of enzyme extract to test tubes containing 1 mL of Tris maleate buffer, the tubes were incubated in water at 37°C for half an hour. TBA reagent (1.5 mL) was then added. The substance in the test tube was heated in boiling water for 10 min and then allowed to cool. Before adding the pyridine/nbutanol solution at a 3:1 (v/v) ratio, the solution was allowed to cool. Next, 1 N sodium hydroxide was added to the test tubes and mixed to stop the reaction. Next, the sample was allowed to stand for 10 min, and the absorbance at 548 nm was measured.
Measurement of H2O2
The previously described method was applied to measure the H2O2 content, although with minor adjustments.[34,35] 10 μL of testis supernatant, 5% dichromate, 0.01 M phosphate buffer (pH 7.0), and glacial acetic acid solution (1:3) were added to each test tube. Each sample was placed in a boiling water bath and boiled for 10 min. After cooling, the OD at 570 nm was determined. The H2O2 concentration was calculated using a standard curve, and the results were expressed as μM/mg protein.
Biometric parameters
Size of testes
The size of the testes of all the rats was measured after the completion of treatment while sacrificing with the help of a measuring scale. The individual value, as well as mean values of the size of testes for each group, was noted. The values were expressed in terms of Mean ± SD.
Testes weight
The testes weight of all the rats was measured after the completion of treatment while sacrificing with the help of a laboratory weighing balance. The individual value, as well as mean values of liver weight for each group, was noted. The values were expressed in terms of Mean ± SD.
Statistical analysis
The mean ± standard deviation was used to express the experimental data. To ascertain the degree of significance between the control and experimental groups, Student’s t-test was utilized. P < 0.05 was considered to indicate statistical significance.
RESULTS
Chronic alcoholism and APAP- induced LC significantly decreased the levels of antioxidant enzymes (SOD and CAT) in testes
SOD
Reactive oxygen species (ROS) are created by regular cellular metabolism in living organisms, and metabolic stress has been shown to boost the production of ROS. Oxidative stress disrupts the normal redox balance of the cell, which causes high levels of ROS production. Together, SOD and CAT are the most important antioxidants in the human body, assisting in reducing the harmful effects of oxidants. SOD, a vital component of the core antioxidant system, produces oxygen and H2O2 from superoxide radicals (O2−), which are produced as a result of oxidative stress. In the present study, compared with the normal control group, the AC group and the LC group exhibited considerably decreased SOD activity [Figure 1].
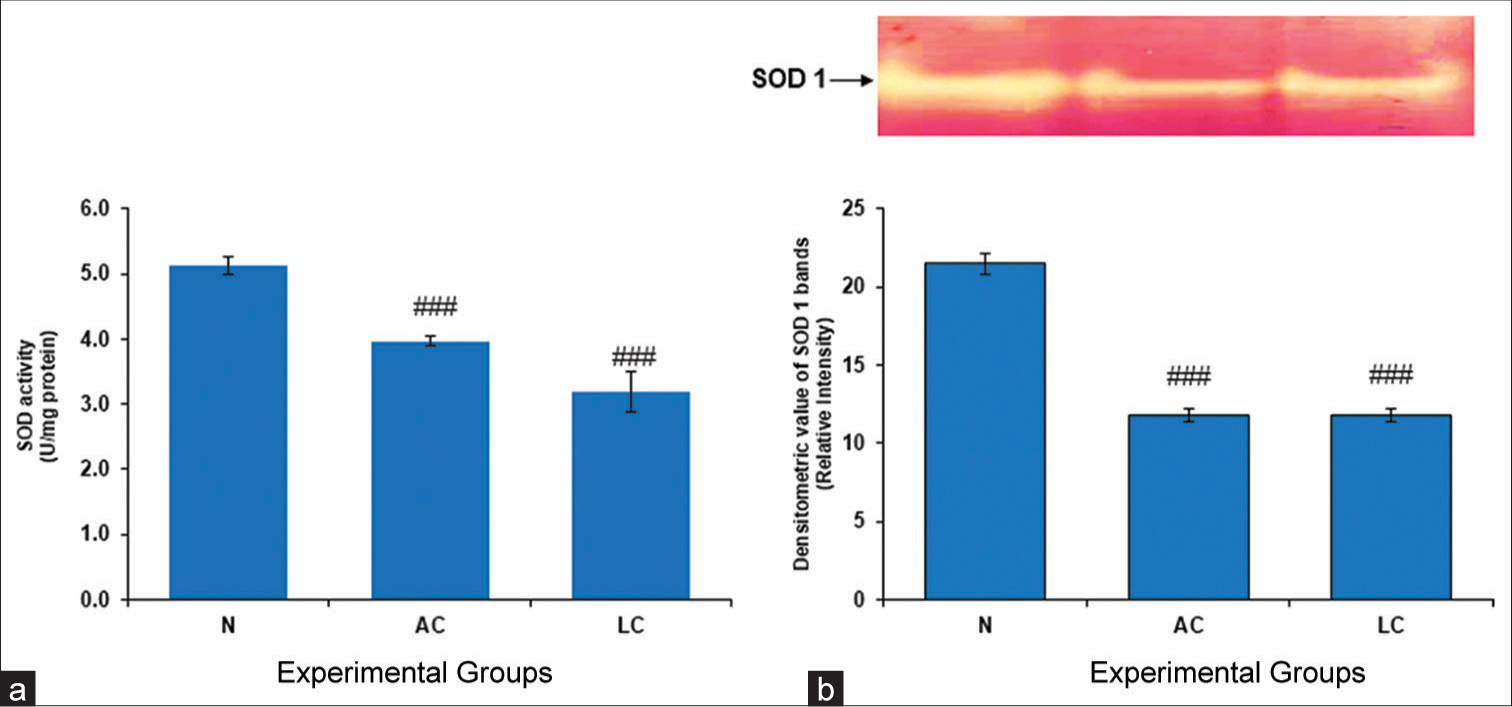
- (a) Superoxide dismutase (SOD) level in the testes of normal, alcohol control (AC) and liver cirrhosis (LC) rats. SOD activity, (b) gel image is representative of SOD activity, performed on 12% native-polyacrylamide gel electrophoresis (PAGE) with 160 μg testes supernatant loaded in each lane followed by substrate-specific development of SOD bands. In the lower panel, relative densitometric values of SOD bands from control and experimental groups have been presented as mean ± standard deviation from three PAGE repeats. ###P < 0.001 (N vs. AC); ###P < 0.001 (N vs. LC) in SOD activity and ###P < 0.001 (N vs. AC); ###P < 0.001 (N vs. LC) in SOD bands, N: Normal.
CAT
The initial line of defense against oxidative stress is provided by downstream enzymes such as CAT and antioxidant enzymes such as SOD. As the activity of these enzymes decreases, the redox state of the cells changes. CAT is an enzyme that converts highly dangerous H2O2 into water. Our findings showed that the groups with AC and LC had much lower levels of CAT activity than did the group with normal liver function [Figure 2].
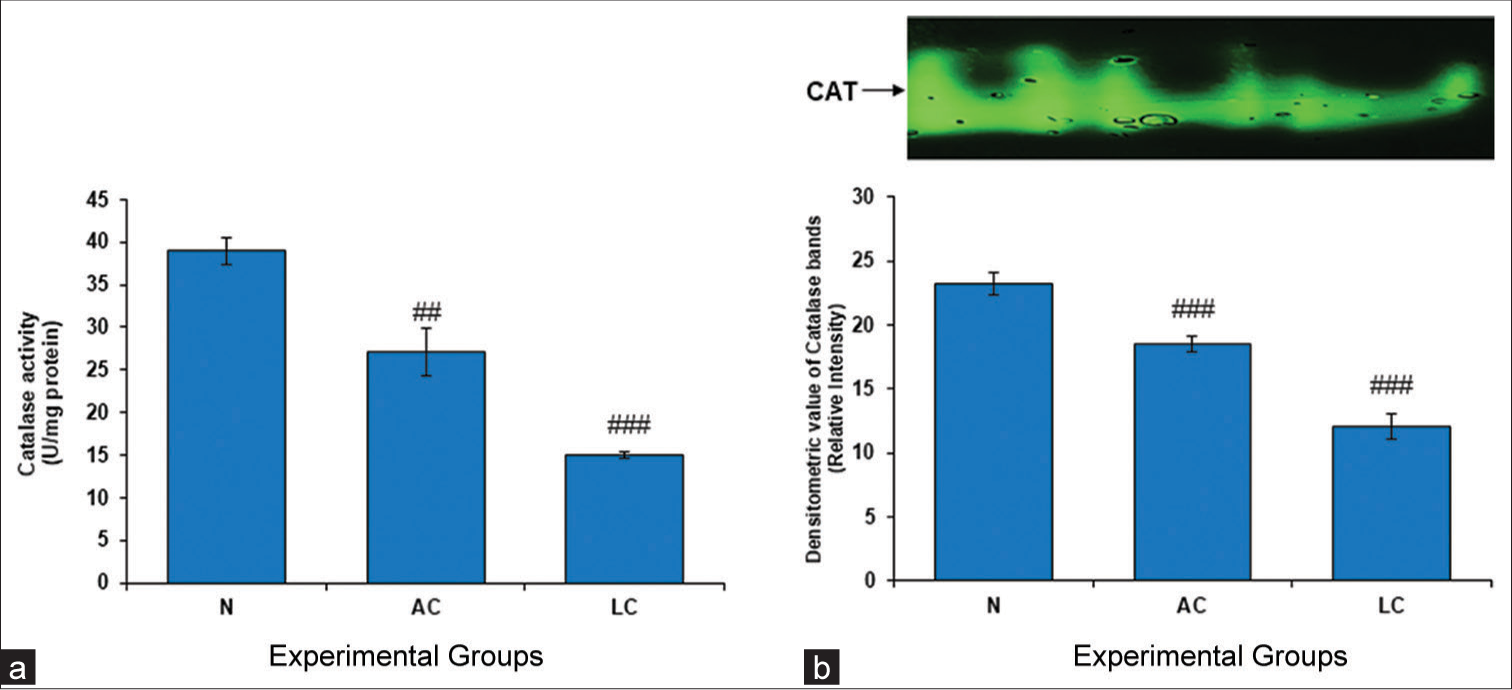
- (a) Catalase (CAT) level in the testes of normal, alcohol control (AC) and liver cirrhosis (LC) rats. CAT activity, (b) gel image is representative of CAT activity, performed on 8% native-polyacrylamide gel electrophoresis (PAGE) with 160 μg testes supernatant loaded in each lane followed by substrate-specific development of CAT bands. In the lower panel, relative densitometric values of CAT bands from control and experimental groups have been presented as mean ± standard deviation from three PAGE repeats. ###P < 0.001 (N vs. AC); ###P < 0.001 (N vs. LC) in CAT activity and ##P < 0.01 (N vs. AC); ###P < 0.001 (N vs. LC) in CAT bands, N: Normal.
Chronic alcoholism and APAP-induced LC significantly increased the level of GST in testes
Mammalian tissues contain an important enzyme called GST, which is responsible for detoxifying reactive metabolites. The present investigation demonstrated a significant increase in GST activity in the testes of AC and LC rats [Figure 3].
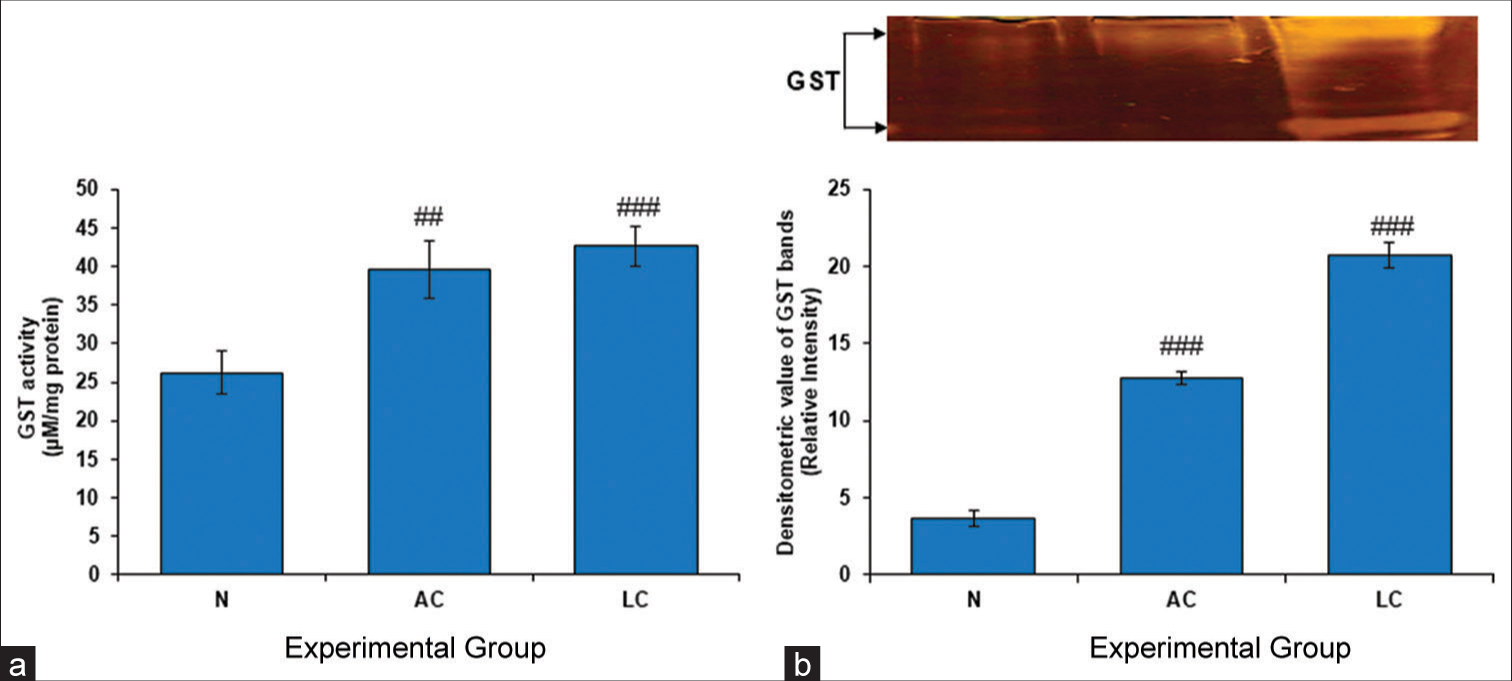
- (a) Glutathione S-transferase (GST) level in the testes of normal, alcohol control (AC) and liver cirrhosis (LC) rats. GST activity, (b) gel image is representative of GST activity, performed on 10% native-polyacrylamide gel electrophoresis (PAGE) with 160 μg testes supernatant loaded in each lane followed by substrate-specific development of GST bands. In the lower panel, relative densitometric values of GST bands from control and experimental groups have been presented as mean ± standard deviation from three PAGE repeats. ###P < 0.001 (N vs. AC); ###P < 0.001 (N vs. LC) in GST activity and ##P < 0.01 (N vs. AC); ### P < 0.001 (N vs. LC) in GST bands, N: Normal.
LC induced by chronic alcoholism and APAP significantly increased the levels of H2O2 and LPO in the testes
Lower cellular free radical scavenging abilities and greater free radical formation during LC metabolism may be connected to the presence of free radical-induced membrane lipids (MDA) and H2O2 in testicular tissue. The present study revealed that the levels of LPO and H2O2 in the testes significantly increased when APAP and alcohol were administered [Figure 4a and b].
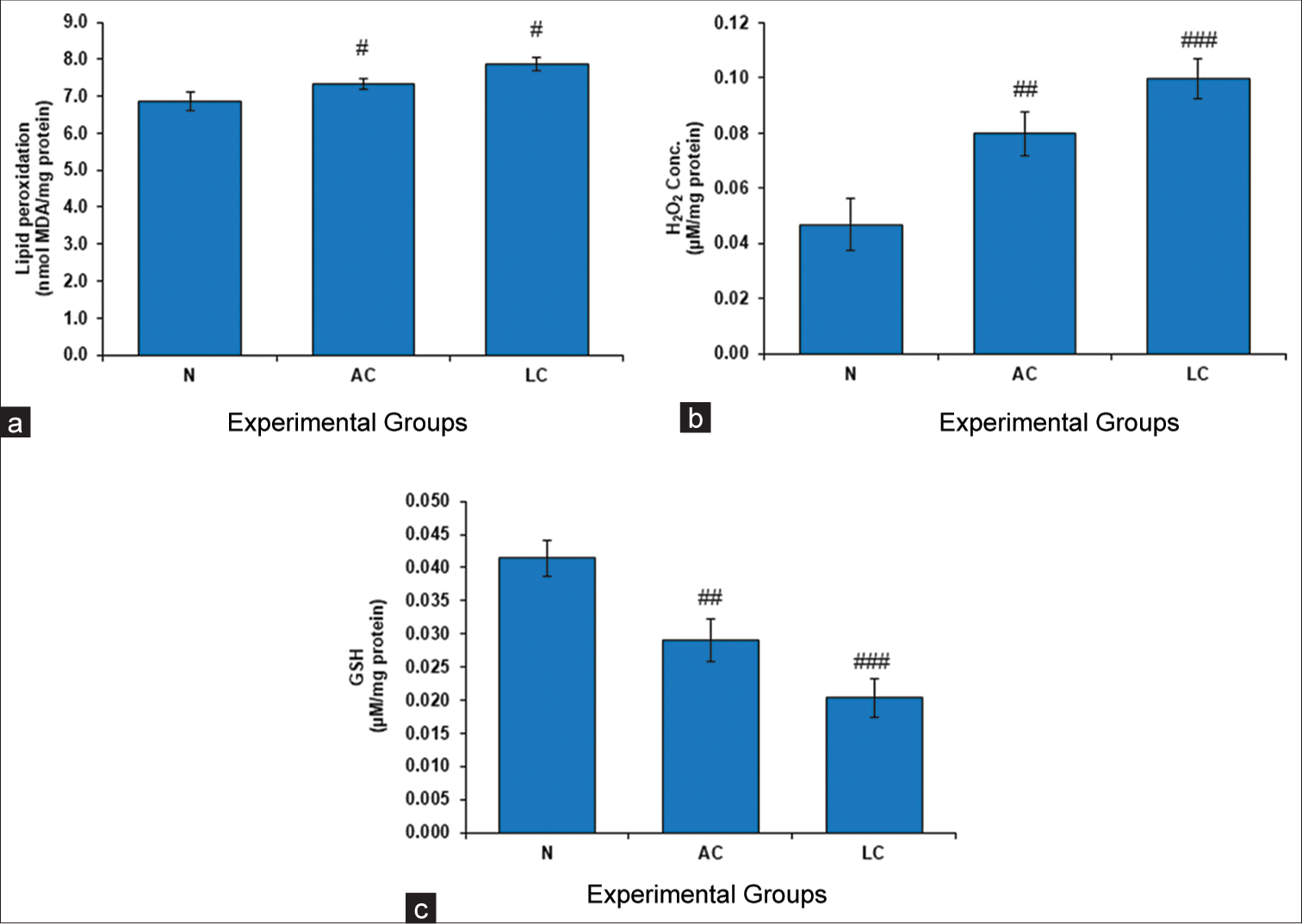
- (a) Level of lipid peroxidation (LPO), (b) Conc. of hydrogen peroxide (H2O2) and (c) level of glutathione (GSH) in testes of normal, liver cirrhosis (LC) and alcohol control (AC) treated rats. Values represent mean ± standard deviation. #P < 0.05 (N vs. AC); #P < 0.05 (N vs. LC) in LPO, ##P < 0.01 (N vs. AC); ###P < 0.01 (N vs. LC) in H2O2 and ##P < 0.01 (N vs. AC); ###P < 0.001 (N vs. LC) in GSH, N: Normal.
GSH
GSH has the ability to protect vital biological components from damage caused by peroxides, lipid peroxides, heavy metals, ROS, and free radicals. Our study revealed that the GSH activity in the AC and LC groups was significantly lower than that in the normal control group [Figure 4c].
LC significantly increased the level of glycolytic enzyme (LDH) in testes
The anaerobic conversion of pyruvic acid to lactic acid and vice versa in the human body depends on the glycolytic enzyme LDH. LDH is a commonly used marker in toxicology and clinical chemistry for assessing damage to cells, tissues, and organs. LDH activity was considerably greater in the LC group and the AC group in the present study than in the normal control group [Figure 5]. Low cellular damage in normal tissue and lower metabolic demand were the two major reasons behind a very low-intensity band of LDH activity in the normal control group.
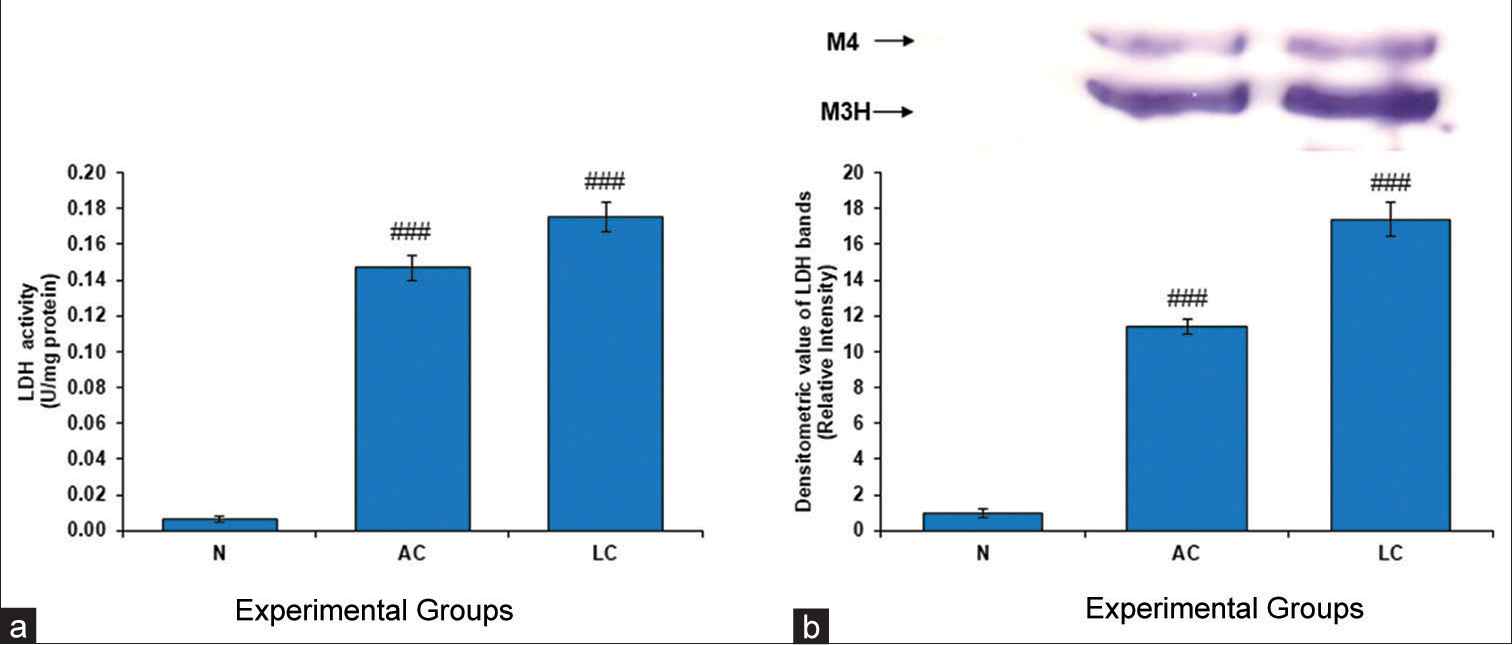
- (a) Lactate dehydrogenase (LDH) level in the testes of normal, alcohol control (AC) and liver cirrhosis (LC) rats. LDH activity, (b) gel image is representative of LDH activity, performed on 10% native-polyacrylamide gel electrophoresis (PAGE) with 160 μg testes supernatant loaded in each lane followed by substrate-specific development of LDH bands. In the lower panel, relative densitometric values of LDH bands from control and experimental groups have been presented as mean ± standard deviation from three PAGE repeats. ###P < 0.001 (N vs. AC); ###P < 0.001 (N vs. LC) in LDH activity and ###P < 0.001 (N vs. AC); ###P < 0.001 (N vs. LC) in LDH bands. N: Normal. M4 is muscle type 4 and M3H is muscle type 3 and heart type 1.
LC significantly decreased the size and weight of testes
The relative size as well as the weight of testes was observed to decrease significantly in both AC and LC-treated groups as compared to the normal group [Figure 6a and b].
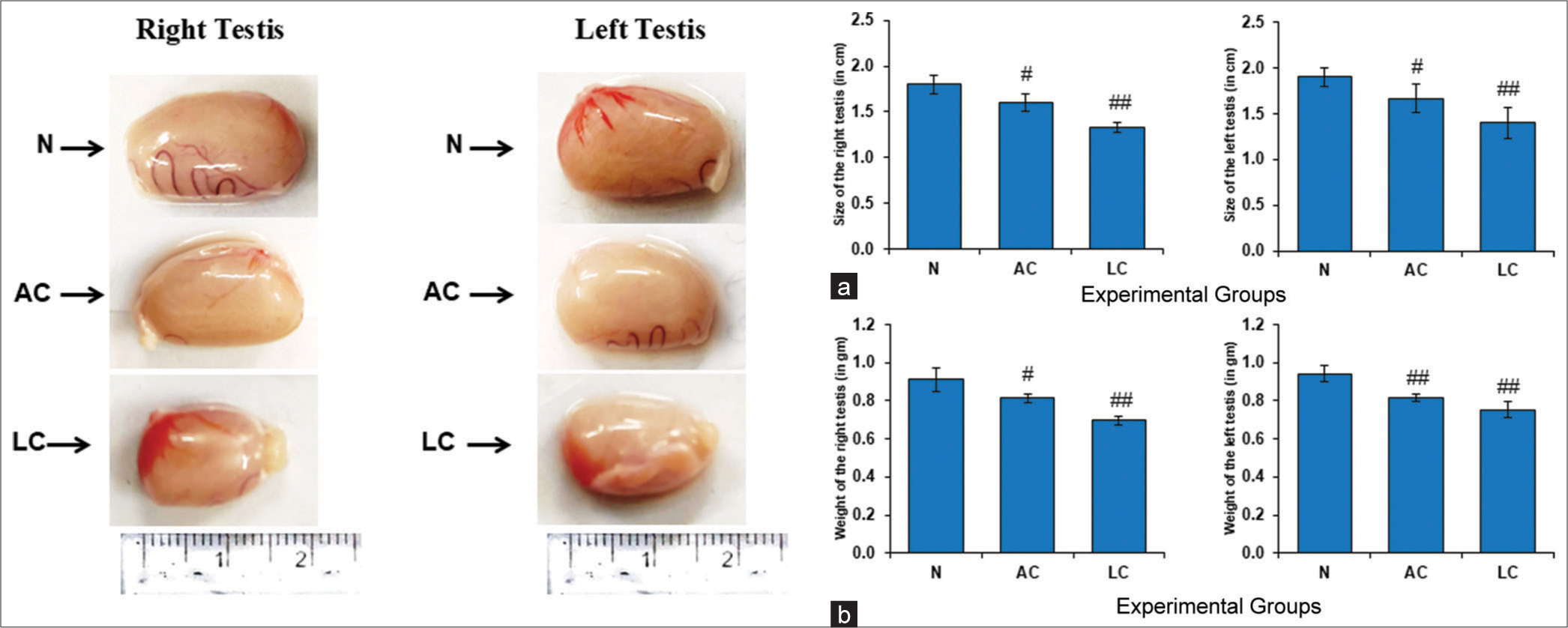
- (a) Size of the testes of normal, alcohol control (AC) and liver cirrhosis (LC) rats (in cm), control and experimental groups have been presented as mean ± standard deviation (SD) from three sets of the testes size (right testis) #P < 0.05 (N vs. AC); ##P < 0.01 (N vs. LC) and (left testis) #P < 0.05 (N vs. AC); ##P < 0.01 (N vs. LC) in testes size. (b) Weight of the testes of normal, AC and LC rats (in g), control and experimental groups have been presented as mean ± SD from three sets of the testes weight (right testis) #P < 0.05 (N vs. AC); ##P < 0.01 (N vs. LC) and (left testis) ##P < 0.01 (N vs. AC); ##P < 0.01 (N vs. LC) in testes weight, N: Normal.
DISCUSSION
After drinking too much alcohol, the liver accumulates acetaldehyde, which activates cytochrome P4502E1 (CYP2E1).[36] ROS are produced when CYP2E1 is activated, which further promotes the synthesis of acetaldehyde.[37] Meanwhile, an increase in the interaction between acetaldehyde and GSH lowers the body’s supply of antioxidants, aggravating liver damage and creating an imbalance between oxidants and antioxidants.[38] Rats administered alcohol, and APAP demonstrated alterations in the levels of antioxidant and glycolytic enzymes in their liver and testicular tissue.[39]
Portal hypertension, which is frequently caused by cirrhosis, can impair the blood supply to the testes and exacerbate testicular dysfunction. Testicular atrophy and gonadal dysfunction are well-known clinical events that can develop in patients with advanced cirrhosis. Testicular atrophy, a medical condition in which the testes shrink and lose their function. Reduced blood flow and hormonal abnormalities are frequently the causes of testicular atrophy. Hypogonadism, a disorder characterized by decreased testosterone levels, can result from cirrhosis. This happens as a result of the liver’s decreased capacity to metabolize hormones, which increases estrogen levels. Abnormal metabolism of trace elements is also present in the testes of cirrhotic rats, which may impact normal spermatogenesis, maturation, and fertilization in cirrhotic rats.[40] On evaluating the testes weight and size, the present study revealed that LC and AC caused the testes to shrink significantly compared to normal testes [Figure 6]. This resulted from advanced cirrhosis-related gonadal dysfunction and testicular atrophy.
Hepatotoxins can produce ROS.[41] Due to the presence of one or more unpaired electrons, these reactive species, which include superoxide, nitric oxide, and lipid peroxyl radicals, are extremely unstable molecules with high reactivity.[26] ROS are produced during normal cellular metabolism and metabolic reprogramming causes their levels to rise in situations when cells are growing and multiplying quickly in a nutritionally deficient environment. ROS production usually causes oxidative stress that impairs the redox balance of an organism. The human body has a highly evolved redox defense mechanism that includes enzymes such as CAT and SOD, which scavenge ROS to counteract their harmful effects. Hepatotoxicity is a major factor in increasing oxidative stress levels, which can alter an individual’s susceptibility to disease. Two criteria have been used to evaluate antioxidant enzymes in this case: the quantitative measurement of the enzymatic activity in cell-free extracts and the quantity of antioxidant enzymes ascertained by an in-gel test based on nondenaturing PAGE analysis. An in-gel-based enzyme detection method was developed and used to ascertain the physiological relevance at the cellular level to obtain an appropriate profile of the enzymatic proteins and their active fractions.[42] In addition, a quantitative assessment of the enzyme activity was carried out to corroborate the PAGE profile results.
SOD, the first antioxidant enzyme in the antioxidant cascade, neutralizes superoxide radicals produced under oxidative stress. SOD is the most important primary antioxidant enzyme because it catalyzes the committed step in the antioxidant pathway. Our study revealed that the groups with LC and the AC group exhibited reduced SOD activity in comparison to the normal control group. The rate at which superoxide was converted to H2O2 increased with increasing SOD levels.
CAT is the second enzyme in the antioxidant pathway that breaks down H2O2 into oxygen and water. Our native PAGE data revealed lower CAT activity in the AC and LC groups than in the control groups. CAT activity often increases in response to stress or disease to remove harmful H2O2. Redox equilibrium is lessened when H2O2 is converted by CAT into oxygen and water.
GSTs, which are phase II detoxification enzymes, are essential for the biotransformation or detoxification of both harmful endogenous and exogenous substances. It also shields against oxidative stress in addition to carcinogens such as nitrosamines and polycyclic aromatic hydrocarbons.[43] A family of enzymes known as GSTs is found in the cytoplasm of cells and is responsible for catalyzing several substitution processes. GSH replaces a group of hepatotoxicants that are easily removed during these processes, averting potentially harmful reactions. In addition, we observed a significant decrease in the majority of antioxidant enzymes during alcohol- APAP-induced toxicity, which was connected to an increase in LPO and H2O2.
The testes of the AC and LC rats exhibited significantly greater levels of GST, which can function as a detoxifying mechanism to eliminate harmful reactive metabolites from hepatotoxicants. Hepatotoxicants metabolize GSH through a process that is facilitated by GST; therefore, it seems obvious that these compounds increase GST activity.
H2O2 and membrane lipids (MDA) in testis tissue may be the result of free radicals generated by enhanced LC metabolism in conjunction with a reduced ability of cells to scavenge free radicals (reduced total thiol). The present study revealed that administering alcohol and APAP to Wistar rats significantly increased LPO and H2O2 levels. The form, fluidity, and function of membranes can be altered by LPO, which can also possibly alter ion flow through its effects on membrane lipids, membrane receptors, and membrane-bound enzymes.[44]
In agreement with this, in an animal model of microcystinLR (MC-LR) toxicity, nitrate and MC-LR together have been reported to increase the degree of toxicological damage and cause a cascade response that boosts the level of LPO in a mouse model.[45] Many enzymes can undergo structural changes and functional deactivation as a result of oxidative protein modification caused by diazinon-induced oxidative stress in the livers of mice.[46] Furthermore, the elevated carbonyl content in proteins resulting from rotenone administration in positive control animals suggests that irreversible protein modification is triggered by oxidative processes.[47] Rat liver tissue exposed to arsenic exhibits increased lipid and protein peroxidation in membranes.[48]
The amount of H2O2 present in cells is often used for determining the extent of oxidative stress and damage at the cellular level. Compared to those in the normal control group, H2O2 levels in the testes were significantly greater in the group administered alcohol and APAP, suggesting that LC caused oxidative stress. H2O2 might be one of the most hazardous species for oxygen metabolism since it can cross membranes.
The present study unequivocally demonstrated that exposure to APAP and alcohol increases the concentration of these ROS. Hepatotoxicants are known to increase ROS production, which leads to oxidative stress in several tissues, particularly cells in the liver and testes.[49] Consistent with our findings, studies have demonstrated that specific micronutrients, including coenzyme Q10, can shield mice from MC-LR-induced toxicity by controlling and restoring H2O2 to normal.[34]
LDH (an oxidoreductase) is a bidirectional enzyme that aids in the conversion of pyruvate to lactate and lactate to pyruvate. Severely injured cells release intracellular LDH into the media very quickly. One common indicator of cell damage is elevated LDH activity. The present investigation revealed that the testes of rats in the normal group had significantly lower LDH isozyme expression than those in the AC and LC groups. It is well known that increased levels of LDH are linked with various diseases [Figure 7].
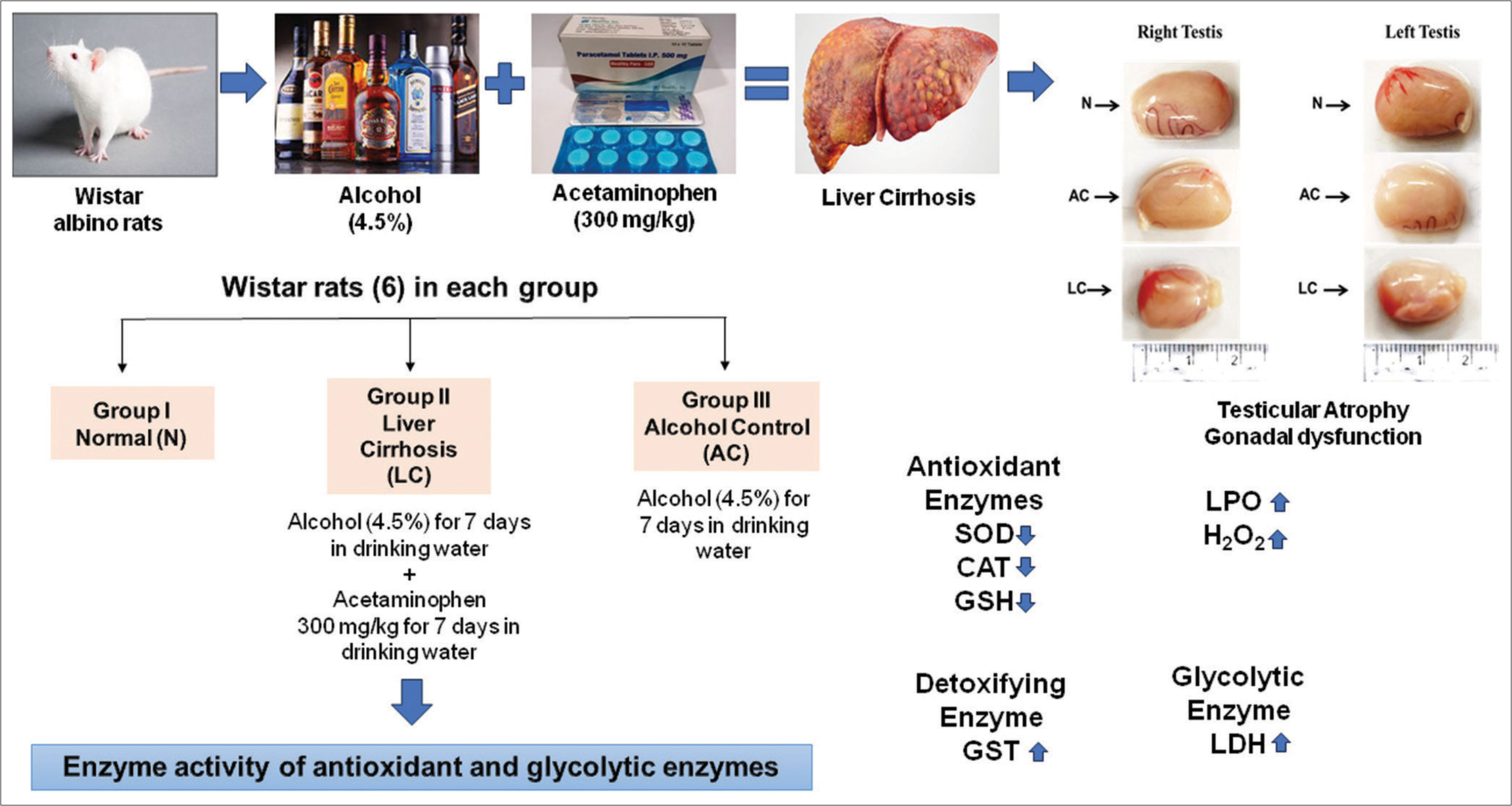
- Brief discussion and summary of the study. N: Normal, AC: Alcohol control, LC: Liver cirrhosis, SOD: Superoxide dismutase, CAT: catalase, GST: glutathione S-transferase, GSH: glutathione, LDH: Lactate dehydrogenase.
CONCLUSION
This is the first study, to the best of our knowledge, that highlighted chronic alcoholism and APAP-induced hepatotoxicity and the induction of LC, further validating its subsequent effect on the testes. Our results appear to be promising for normalizing the enzyme activity of antioxidant pathways that are altered during hepatotoxicity. Furthermore, the key enzyme of the glycolytic pathway, LDH, was also evaluated. This is the first report of the development of testicular atrophy of the testes in patients with chronic alcoholism and APAP-induced LC. The findings from the present study are expected to provide in-depth insight into the mechanism of action between testicular atrophy and LC.
Highlights
Liver cirrhosis was induced by chronic alcoholism and acetaminophen in the murine model.
Testicular atrophy and gonadal dysfunction were induced due to liver cirrhosis.
Due to the induction of liver cirrhosis, ROS-mediated alterations in antioxidant enzymes were observed in testes.
An enzyme of the glycolytic pathway (LDH) is also altered in testes due to liver cirrhosis.
Acknowledgment
Debabrata Dash is grateful to ICMR and UGC for the fellowship. The authors are thankful to the Department of Zoology, Dr. Harisingh Gour Vishwavidyalaya (A Central University), Sagar, and the DST-FIST program (SR/FST/LS 1/2018/176(C)) to the Department of Zoology for providing infrastructural facilities.
Ethical approval
The research/study was approved by the Institutional Review Board at Dr. Harisingh Gour Vishwavidyalaya Institutional Animal Ethics Committee, number 379/CPCSEA/IAEC-2021/009, dated December 14, 2021.
Declaration of patient consent
Patient consent was not required as there are no patients in this study.
Conflicts of interest
There are no conflicts of interest.
Use of artificial intelligence (AI)-assisted technology for manuscript preparation
The authors confirm that there was no use of artificial intelligence (AI)-assisted technology for assisting in the writing or editing of the manuscript and no images were manipulated using AI.
Financial support and sponsorship
This study was financially supported by a project from DSTSERB (CRG/2018/003780) and DBT-BUILDER (BT/INF/22/SP47619/2022), Govt. of India, sanctioned to Raj Kumar Koiri and Indian Council of Medical Research (ICMR), New Delhi, India (No.3/1/2(10)/CD/2022-NCD-II) to Debabrata Dash.
References
- Mammalian testes: Structure and function. In: Introduction to mammalian reproduction. New York: Springer; 2003. p. :1-19.
- [CrossRef] [Google Scholar]
- Liver cirrhosis in Malaysia: Peculiar epidemiology in a multiracial Asian country. J Gastroenterol Hepatol. 2011;26:1333-7.
- [CrossRef] [PubMed] [Google Scholar]
- Something new in liver cirrhosis epidemiology. Lancet. 1989;2:395-6.
- [CrossRef] [PubMed] [Google Scholar]
- Diagnosis and treatment of alcohol-associated liver diseases: 2019 Practice guidance from the American association for the study of liver diseases. Hepatology. 2020;71:306-33.
- [CrossRef] [PubMed] [Google Scholar]
- MAFLD: A consensus-driven proposed nomenclature for metabolic associated fatty liver disease. Gastroenterology. 2020;158:1999-2014.e1.
- [CrossRef] [PubMed] [Google Scholar]
- The role of vascular injury and congestion in the pathogenesis of cirrhosis: The congestive escalator and the parenchymal extinction sequence. Curr Hepatol Rep. 2020;19:40-53.
- [CrossRef] [Google Scholar]
- Resolving the fibrotic niche of human liver cirrhosis at single-cell level. Nature. 2019;575:512-8.
- [CrossRef] [PubMed] [Google Scholar]
- Nitric oxide as a mediator of hemodynamic abnormalities and sodium and water retention in cirrhosis. N Engl J Med. 1998;339:533-41.
- [CrossRef] [PubMed] [Google Scholar]
- The liver may act as a firewall mediating mutualism between the host and its gut commensal microbiota. Sci Transl Med. 2014;6:237ra66.
- [CrossRef] [PubMed] [Google Scholar]
- Bacterial infections in cirrhosis: A position statement based on the EASL special conference 2013. J Hepatol. 2014;60:1310-24.
- [CrossRef] [PubMed] [Google Scholar]
- The effects of paracetamol on the liver and kidney functions of a rat model following prolonged alcohol administration. J Drug Metab Toxicol. 2021;12:256.
- [Google Scholar]
- Paracetamol, alcohol and the liver. Br J Clin Pharmacol. 2000;49:291-301.
- [CrossRef] [PubMed] [Google Scholar]
- Insulin-like growth factor-I reverts testicular atrophy in rats with advanced cirrhosis. Hepatology. 2000;31:592-600.
- [CrossRef] [Google Scholar]
- Dysbalanced sex hormone status is an independent predictor of decompensation and mortality in patients with liver cirrhosis. Hepatol Res. 2019;49:201-11.
- [CrossRef] [PubMed] [Google Scholar]
- Conversion of androgens to estrogens in cirrhosis of the liver. J Clin Endocrinol Metab. 1975;40:1018-26.
- [CrossRef] [PubMed] [Google Scholar]
- Sexual dysfunctions and their treatment in liver diseases. World J Hepatol. 2022;14:1530-40.
- [CrossRef] [PubMed] [Google Scholar]
- Liver transplantation and quality of life: Relevance of a specific liver disease questionnaire. Liver Int. 2008;28:99-106.
- [CrossRef] [PubMed] [Google Scholar]
- Erectile dysfunction in compensated liver cirrhosis. Dig Liver Dis. 2019;51:843-9.
- [CrossRef] [PubMed] [Google Scholar]
- Male sexual disturbances in liver diseases: What do we know? J Endocrinol Invest. 2010;33:501-5.
- [CrossRef] [PubMed] [Google Scholar]
- Sexual dysfunction after liver transplantation. Liver Transpl. 2009;15:S50-6.
- [CrossRef] [PubMed] [Google Scholar]
- Prevalence and risk factors of erectile dysfunction in patients with liver cirrhosis: A systematic review and meta-analysis. Hepatol Int. 2023;17:452-62.
- [CrossRef] [PubMed] [Google Scholar]
- Protein measurement with the Folin phenol reagent. J Biol Chem. 1995;193:265-75.
- [CrossRef] [Google Scholar]
- Regression of Dalton's lymphoma in vivo via decline in lactate dehydrogenase and induction of apoptosis by a ruthenium (II)-complex containing 4-carboxy N-ethylbenzamide as ligand. Invest New Drugs. 2009;27:503-16.
- [CrossRef] [PubMed] [Google Scholar]
- Superoxide dismutase: Improved assays and an assay applicable to acrylamide gels. Anal Biochem. 1971;44:276-87.
- [CrossRef] [PubMed] [Google Scholar]
- Protective and therapeutic effects of sildenafil and tadalafil on aflatoxin B1-induced hepatocellular carcinoma. Mol Cell Biochem. 2021;476:1195-209.
- [CrossRef] [PubMed] [Google Scholar]
- Study on glutathione S-transferase (GST) inhibition assay by triclabendazole. I: Protoscoleces (hydatid cyst; Echinococcus granulosus) and sheep liver tissue. Iran J Public Health. 2005;34:38-46.
- [Google Scholar]
- Metal Cu (II) and Zn (II) bipyridyls as inhibitors of lactate dehydrogenase. Biometals. 2008;21:117-26.
- [CrossRef] [PubMed] [Google Scholar]
- Ameliorative effect of piracetam on emamectin benzoate induced perturbations in the activity of lactate dehydrogenase in murine system. Adv Redox Res. 2021;3:100019.
- [CrossRef] [Google Scholar]
- Disc electrophoresis. II. Method and application to human serum proteins. Ann N Y Acad Sci. 1964;121:404-27.
- [CrossRef] [PubMed] [Google Scholar]
- SIRT1-mediated amelioration of oxidative stress in kidney of alcohol-aflatoxin-B1-induced hepatocellular carcinoma by resveratrol is catalase dependent and GPx independent. J Biochem Mol Toxicol. 2020;34:e22576.
- [CrossRef] [PubMed] [Google Scholar]
- Estimation of product of lipid peroxidation (malonyl dialdehyde) in biochemical systems. Anal Biochem. 1996;16:359-64.
- [CrossRef] [PubMed] [Google Scholar]
- Amelioratory effect of coenzyme Q10 on potential human carcinogen microcystin-LR induced toxicity in mice. Food Chem Toxicol. 2017;102:176-85.
- [CrossRef] [PubMed] [Google Scholar]
- Pathophysiological aspects of alcohol metabolism in the liver. Int J Mol Sci. 2021;22:5717.
- [CrossRef] [PubMed] [Google Scholar]
- Role of oxidative stress in alcohol-induced liver injury. Arch Toxicol. 2009;83:519-48.
- [CrossRef] [PubMed] [Google Scholar]
- A comparative study of plant and animal mitochondria exposed to paraquat reveals that hydrogen peroxide is not related to the observed toxicity. Toxicol In Vitro. 2004;18:733-9.
- [CrossRef] [PubMed] [Google Scholar]
- Integrated application of transcriptomics and metabonomics yields new insight into the toxicity due to paracetamol in the mouse. J Pharm Biomed Anal. 2004;35:93-105.
- [CrossRef] [PubMed] [Google Scholar]
- Trace element levels in the testes of thioacetamide-induced cirrhotic rats. J Trace Elem Exp Med. 2001;14:383-92.
- [CrossRef] [Google Scholar]
- Contaminant-stimulated reactive oxygen species production and oxidative damage in aquatic organisms. Mar Pollut Bull. 2001;42:656-66.
- [CrossRef] [PubMed] [Google Scholar]
- Moderate grade hyperammonemia induced concordant activation of antioxidant enzymes is associated with prevention of oxidative stress in the brain slices. Neurochem Res. 2012;37:171-81.
- [CrossRef] [PubMed] [Google Scholar]
- Modulation of antioxidant enzymes, SIRT1 and NF-κB by resveratrol and nicotinamide in alcohol-aflatoxin B1-induced hepatocellular carcinoma. J Biochem Mol Toxicol. 2021;35:e22625.
- [CrossRef] [PubMed] [Google Scholar]
- Effect of combined exposure of commonly used organophosphate pesticides on lipid peroxidation and antioxidant enzymes in rat tissues. Pestic Biochem Phys. 2011;99:148-56.
- [CrossRef] [Google Scholar]
- Nitrate enhances microcystin-LR induced toxicity in mice. Austin J Mol Cell Biol. 2015;2:1006.
- [CrossRef] [PubMed] [Google Scholar]
- Amelioratory effect of vitamin E on organophosphorus insecticide diazinon-induced oxidative stress in mice liver. Pestic Biochem Phys. 2010;96:101-7.
- [CrossRef] [Google Scholar]
- Catechin, quercetin and taxifolin improve redox and biochemical imbalances in rotenone-induced hepatocellular dysfunction: Relevance for therapy in pesticide-induced liver toxicity? Pathophysiology. 2018;25:365-71.
- [CrossRef] [PubMed] [Google Scholar]
- Toxic effects of imidacloprid combined with arsenic: Oxidative stress in rat liver. Toxicol Ind Health. 2018;34:726-35.
- [CrossRef] [PubMed] [Google Scholar]
- Chlorpyrifos induced alterations in the levels of hydrogen peroxide, nitrate and nitrite in rat brain and liver. Pestic Biochem Phys. 2009;94:55-9.
- [CrossRef] [Google Scholar]