Translate this page into:
Control of sperm quality in the epididymis by disintegration and removal – A transmission electron microscopy study of abnormal sperm of aflatoxin-treated rat
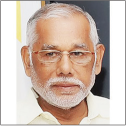
*Corresponding author: Mohammad Abdulkader Akbarsha, Ph.D., Department of Biotechnology, National College (Autonomous), Tiruchirappalli, India. akbarbdu@gmail.com
-
Received: ,
Accepted: ,
How to cite this article: Faisal K, Akbarsha MA. Control of sperm quality in the epididymis by disintegration and removal – A transmission electron microscopy study of abnormal sperm of aflatoxin-treated rat. J Reprod Healthc Med. 2024;5:4. doi: 10.25259/JRHM_23_2023
Abstract
Objectives:
The mammalian epididymis is a dynamic organ endowed with diverse roles, including sperm quality control, which appears to occur during the epididymal processing and storage to prevent the misshapen spermatozoa from being present in the ejaculate in large numbers. This quality control mechanism is capable of identifying and removing dead/defective spermatozoa. In this respect, the role of a dense matrix arising from epididymal secretory aposomes/epididymosomes and associated disintegration/dissolution of dead/defective spermatozoa has been reported. This study was conceived to find if the mechanistic details of the disintegration of the parts of the spermatozoa along the length encompass the same or different patterns.
Material and Methods:
We used photomicrographs from the experimental rat model induced into defective/unviable spermatozoa by aflatoxin B1 treatment.
Results:
A meticulous screening of the matrix-embedded spermatozoa reflected different courses of disintegration of the major parts, namely, head, mid-piece, and flagellum. The disintegration of the head began with swelling of the acrosome and its lysis, followed by the gradual disintegration of the nucleus. In the mid-piece, the plasma membrane underwent disintegration first, and this was followed by disorganization and disintegration of the mitochondrial sheath and, subsequently, the outer dense fibers (ODFs). In the flagellum, neither the plasma membrane nor the fibrous sheath (FS) indicated any trace of disintegration initially. Rather, the FS and the plasma membrane were lifted off from the ODF-axoneme complex and, subsequently, with the FS remaining intact, the ODFs and the axonemal doublets on one side disintegrated, followed by disintegration of the remaining ODFs and the axonemal doublets.
Conclusion:
In the disintegration process, the spermatozoa’s head, mid-piece, and flagellum follow different courses, reflecting on the respective structural compositions.
Keywords
Abnormal spermatozoa
Sperm quality control
Sperm disintegration
Epididymosomes
Dense matrix
INTRODUCTION
The mammalian epididymis is a dynamic organ endowed with diverse roles concerning the post-testicular physiological maturation of spermatozoa and their storage before ejaculation. Sperm quality control is one such role, which appears to occur during epididymal processing and storage to circumvent misshapen, genetically abnormal, or infertile spermatozoa entering the ejaculate in abundance.[1,2] This function of the epididymis has been hypothesized since the days of Simeone and Young[3] and has more recently been substantiated.[4-6] It is well known that many spermatozoa in normal physiological conditions are defective and/or unviable,[7] and sperm abnormalities in men have received extensive scrutiny.[8] Problems in spermatozoa are induced by aberrations in spermatogenesis in the testis (primary defects) or by extragonadal organs, particularly the epididymis (secondary defects).[9,10] There are multiple causes, including toxic insults, that contribute to the genesis of spermatozoa with aberrant morphologies and/or reduced viability ensuing from issues in spermatogenesis, spermiogenesis, and/or epididymal processing.[11,12]
It is enunciated that the more proximal parts of the epididymis, namely, caput and corpus, have a higher incidence of dead and decapitated spermatozoa than the cauda and the ductus deferens.[2] According to Orgebin-Crist,[13] the epididymis reabsorbs at least 50% of spermatozoa released from the testis. These observations imply the existence of a sperm quality control mechanism in the mammalian epididymis, as a matter of routine, even in normal animals[2] or when sperm death signals predominate.[1] This mechanism is capable of identifying and removing dead or defective spermatozoa. Men who undergo vasectomy also experience the disintegration of spermatozoa,[14] and animals that breed seasonally do as well after the mating season.[15,16] In this respect, we have reported the dense matrix-associated disintegration/dissolution of dead/defective spermatozoa.[17] Further, we also traced the source of this dense matrix to the epididymal epithelial principal cell-derived aposomes and the epididymosomes therein.[18] The secretion of the dense material that segregates the sperm cargo for complete disintegration and liquefaction safeguards the healthy/viable spermatozoa from the onslaught by the free radicals. In this context, we developed an interest in finding if the mechanistic details of the disintegration encompass the same pattern along the entire sperm or if the different parts respond differently to the disintegration/dissolution process. In this investigation, we used the photomicrographs from an experimental rat model induced into defective/unviable spermatozoa by aflatoxin B1 (AFB1) treatment mentioned vide supra[17,18] to trace the pattern of disintegration/dissolution.
MATERIAL AND METHODS
The animal study has been reported[17,18], and the Institutional Animal Ethics Committee approved the experiment. Briefly, male Wistar strain 90-day-old rats were raised from a stock obtained from the Indian Institute of Science, Bangalore, India, and fed on an aflatoxin-free pellet diet from Sai Durga Feeds and Foods, Bangalore, India, and water ad libitum. AFB1 was purchased from Sigma Chemical Company (St Louis, MO, USA). The toxin was dissolved in a minimum volume of ethanol and then diluted in olive oil. The preparation was administered to 10 rats at a daily dose of 20 μg/kg body weight for 55 days, the length of one spermatogenic cycle.[19] The route of administration was intramuscular.[17,18,20,21] An equal number of control rats were treated with the vehicle. After being perfused with Karnovsky’s[22] fluid, thin slices of the various segments of epididymis were collected from control and treated rats and fixed in 2.5% glutaraldehyde in cacodylate buffer followed by post-fixation in 1% osmium tetroxide.[23] The tissue slices were thoroughly washed in the buffer, dehydrated in ethanol, cleared in propylene oxide, and embedded in thin viscosity resin (Araldite CY212, SPI-Chem™, Switzerland). Toluidine blue-O was used to stain the semi-thin sections (1 μm thick) cut in a Leica (Jena, Germany) ultramicrotome for examination in a Carl Zeiss (Jena, Germany) research microscope. A CCD camera (Sony, Tokyo, Japan) equipped with the Axiovision (Carl Zeiss, Germany) software was used to capture photomicrographs. From the semithin sections, the regions of interest were selected and cut into ultrathin sections using the same microtome. Uranyl acetate (6%) and lead citrate (0.1%) were used to stain the ultrathin sections for analysis, adopting a transmission electron microscope (Phillips 201C; Amsterdam, the Netherlands). Special attention was given to sperm that were embedded in a dense matrix and underwent dissolution and/or disintegration.[17,18]
RESULTS
The lumen of the ductus epididymidis of aflatoxin-treated rats contained spermatozoa with varied abnormalities, as reported earlier.[20,21,24] These misshapen spermatozoa were found entangled in a dense matrix, resulting from the aposomes that contained the epididymosomes in the lumen of caput epididymidis onwards. These spermatozoa were in different phases of disintegration and/or dissolution.[17,18] A meticulous screening of the matrix-embedded spermatozoa reflected different courses of disintegration of the major parts of the spermatozoa, namely, head, mid-piece, and flagellum.
In the head, the earliest change was swelling of the acrosome, resulting in the appearance of a wider than normal perinuclear space [Figure 1a-c]. This was followed by the collapse of the acrosome, exposing the nucleus [Figure 1d and e]. Subsequently, the homogenous electron-dense nucleus became heterogeneous, with the introduction of clear spaces due to the disintegration of chromatin in a gradual manner [Figure 1f-i]. Thus, the disintegration of the head began with swelling of the acrosome and its lysis, followed by gradual disintegration of the nucleus.
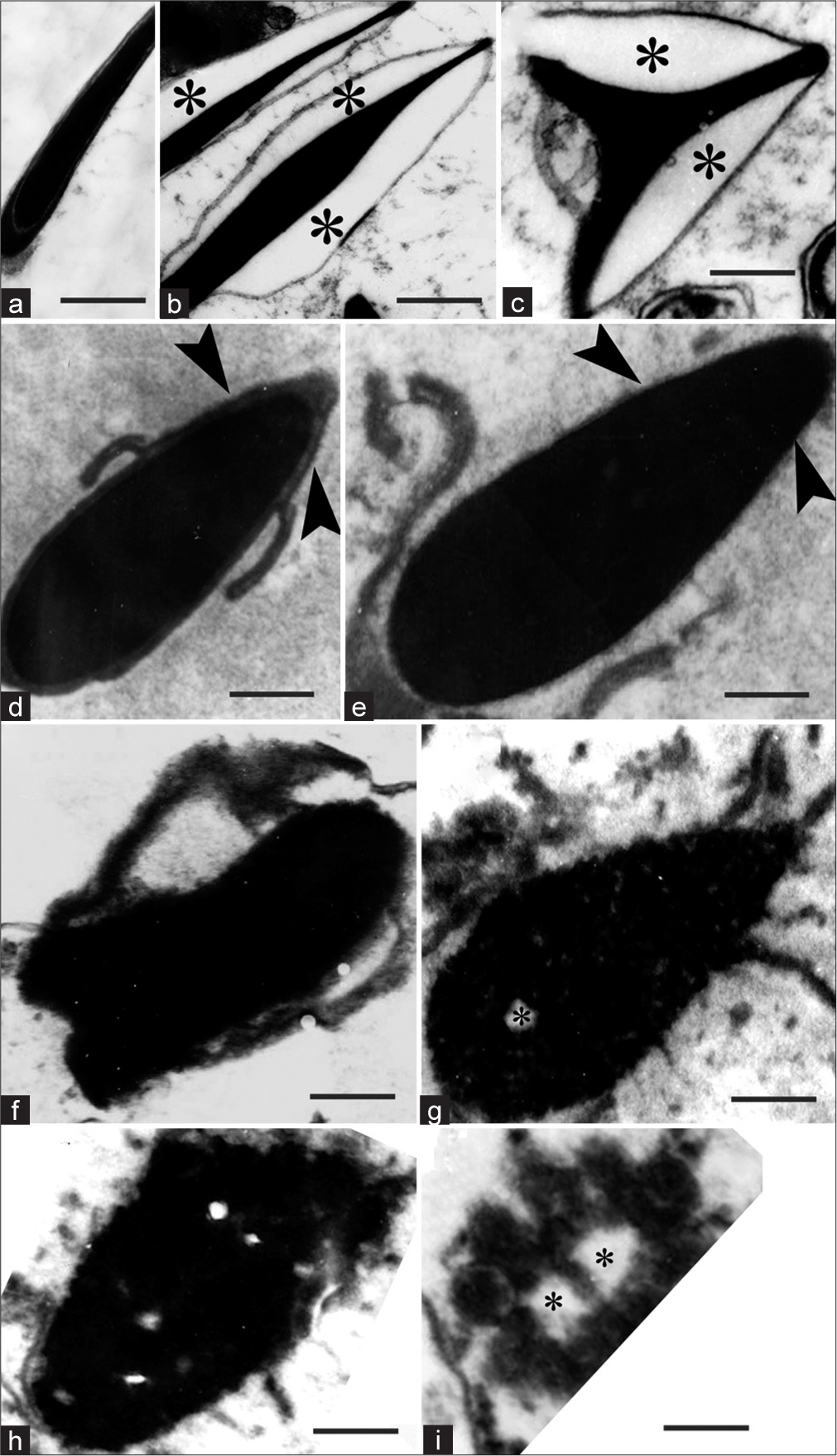
- (a) Transmission electron micrograph (TEM) of longitudinal section of control sperm head. (b–i), TEM of matrix-embedded spermatozoa. (b) Longitudinal Section (LS) of the head; (c) Transverse section of the head (asterisks indicate swelling of acrosome and widening of perinuclear space). (d and e) show collapse of acrosome and exposure of the nucleus (arrowheads). (f-i) Show gradual disintegration of the sperm nucleus (asterisks indicate empty spaces in the nucleus). Scale bar a, b 1.5 μm, c 0.9 μm, d 0.8 μm, e–i 0.6 μm.
In the mid-piece, the earliest change was the disintegration of the plasma membrane, and is very conspicuous compared to the control [Figure 2a and b]. This was followed by disorganization of the mitochondrial sheath, with the latter becoming disintegrated starting from one weak point [Figure 2a-d]. The outer dense fibers (ODFs) took to either of the two following courses. First, they were disorganized and lost their connection with the axoneme [Figure 2e]. Subsequently, the ODFs underwent slow disintegration such that in some sections, there was no trace of ODFs on one side, but those on the other side were intact. Thereafter, the disintegration continued in an irregular pattern with respect to the ODFs [Figure 2f]. Second, it was interesting to see sperm mid-piece sections without any trace of the plasma membrane, mitochondrial sheath, and even axoneme in some cases, leaving only the ODFs intact [Figure 2g-i], which also subsequently disintegrated [Figure 2j-l]. With further disintegration, the sections were not revealing identity as belonging to sperm mid-piece.
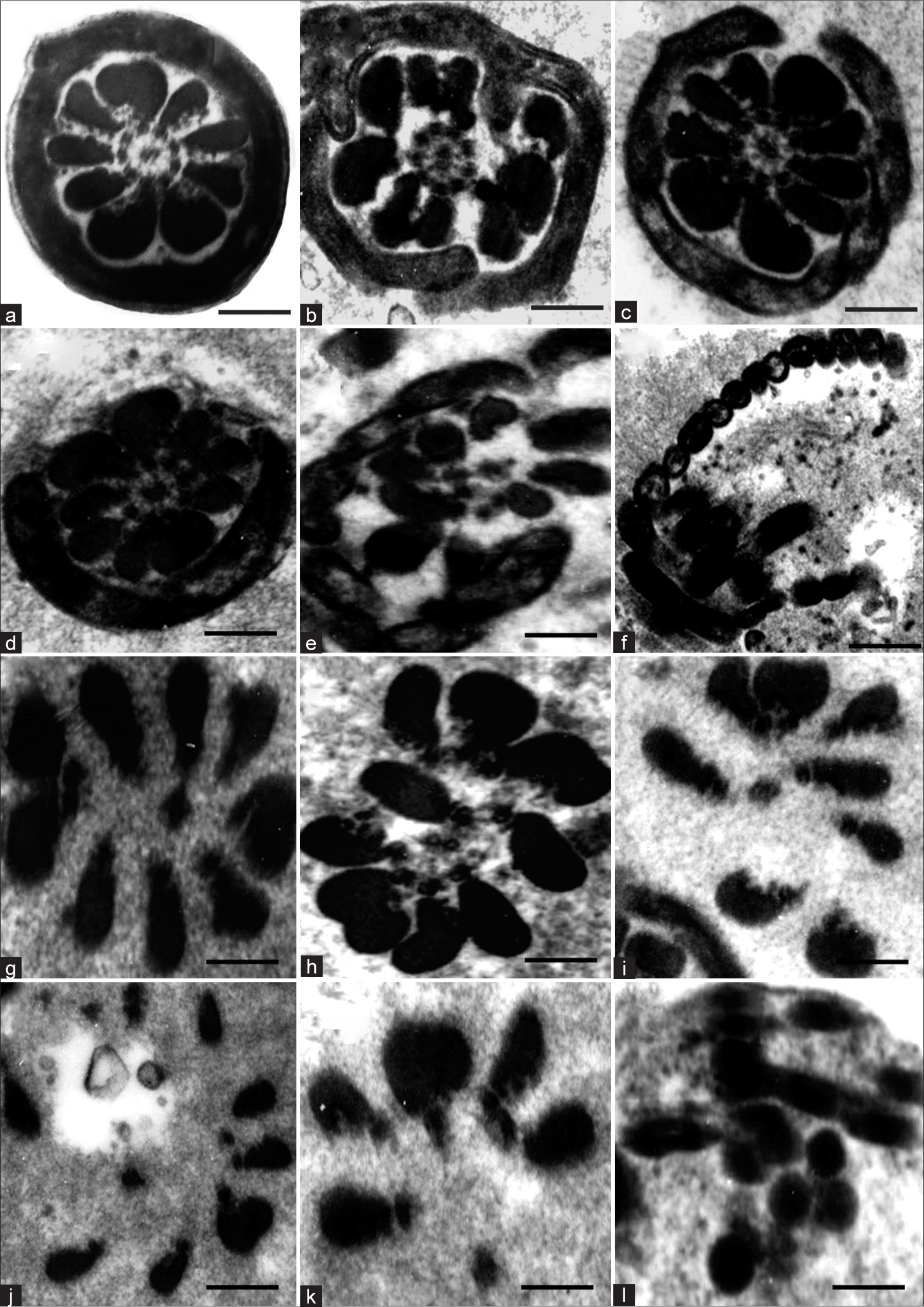
- (a) Transmission electron micrograph (TEM) of Transverse section (TS) of mid-piece of control sperm. (b–l) TEM of mid-piece of matrix-embedded spermatozoa in disintegration, showing the tentative sequence of changes. (g–l) Suggests that outer dense fibers are rather resistant to disintegration. Scale bar a–e 0.3 μm, f 0.4 μm, g-l 0.25 μm.
In the flagellum, neither the plasma membrane nor fibrous sheath (FS) indicated any trace of disintegration initially. Rather, the FS and the plasma membrane were lifted off from the ODFs-axoneme complex [Figure 3a and b]. Subsequently, with the FS remaining intact, the ODFs and the axonemal doublets on one side disintegrated [Figure 3c and d], and later, the remaining ODFs and the axonemal doublets also disintegrated [Figure 3e], as a result of which the principal piece in transverse sections (TSs) appeared as empty vesicles [Figure 3f]. In some TSs of spermatozoa, the ODFs on one side were missing, but such missing ODFs were found outside the FS, that is, between the FS and sperm plasma membrane [Figure 3f]. These are not to be mistaken for matrix-embedded spermatozoa. These are spermatozoa, the flagellum of which was folded, and the folds were fused such that it looks as if there were two axonemes in a common cytoplasm [Figure 3g and h]. These correspond to spermatozoa in which the ODFs were extruded, as reported earlier.[24]
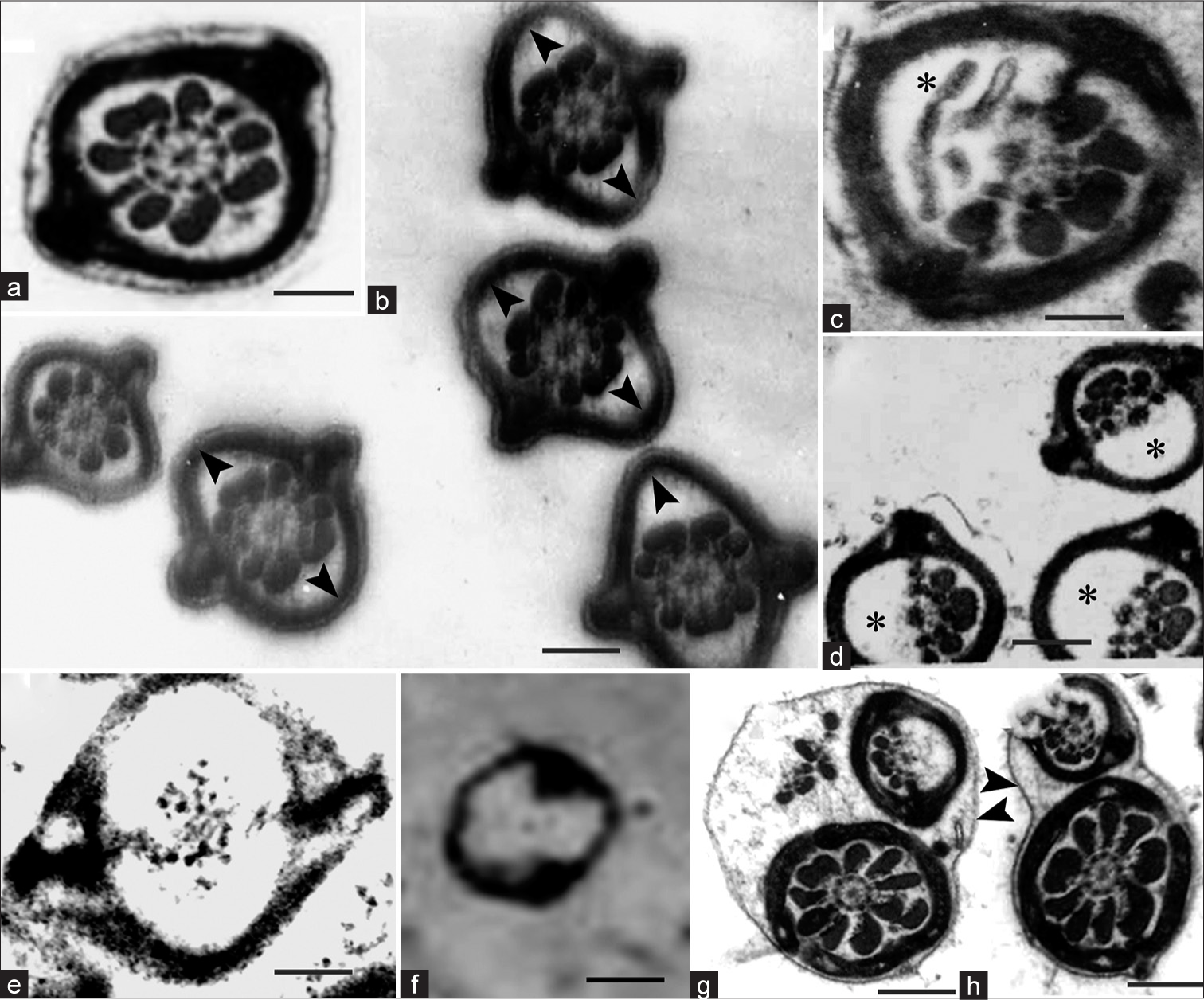
- (a) Transmission electron micrograph (TEM) of Transverse section (TS) of principal piece of control sperm. (b–h) TEM of TS of principal piece of flagellum of matrix-embedded spermatozoa in disintegration. (b) fibrous sheath (FS) is lifted off (arrowheads) from the axoneme-outer dense fiber (ODF) complex; (c) one such spermatozoon magnified (asterisks show disintegrating ODFs); (d) complete disintegration of the ODFs on one side (asterisks); (e) complete disintegration of the ODFs on both sides; (f) complete disintegration of the ODFs and axoneme indicating that FS is more resistant to the disintegration. (g and h) TEM of sperm flagellum, which is folded and fused, could be mistaken for matrix-embedded spermatozoa. Note the intact plasma membranes (arrowhead). Scale bar a, 0.6 μm, b, d, f–h, 0.8 μm, c, e, 0.4 μm.
DISCUSSION
This study reveals that while the dead/defective spermatozoa undergo disintegration and liquefaction while being entangled in a dense material of epididymal lumen, the head, mid-piece, and flagellum of the spermatozoa take different courses in the disintegration process. As regards the sequence of dissolution of spermatozoa, to the best of our knowledge, none of the earlier authors traced it to the stage in this study. In the head, the earliest response was seen in the acrosome, which initially swells and then collapses such that the perinuclear theca is disposed of. Once exposed, the nucleus should be responding to the DNases and proteinases resulting in complete disintegration of the nucleus. According to Jones[1], disposing of the condensed sperm head is more difficult. Trypsinization (to cause decapitation and dissolution of the perinuclear theca) in the presence of a reducing agent, such as dithiothreitol, is an efficient method to decondensed sperm heads in vitro. It is conceivable that sperm heads might start to decondense and degrade in the presence of active proteinases and DNases if enough reducing activity (such as glutathione) is present in the epididymal fluid, and over a few days or weeks, they would eventually disappear.[1] In favor of this conjecture, active DNase and a variety of other nucleolytic enzymes were observed in bull, boar, rabbit, and rat epididymis.[1,25] Perhaps, Jones[1] is justified in stating that disposal of the resistant sperm head, mitochondria, FS, and ODFs would be a problem.
In the mid-piece, the plasma membrane appears to be easily susceptible to the disintegration process, thus exposing the mitochondrial sheath, which then succumbs to the disintegration. The axoneme is the last to disintegrate, indicating the resistance of the ODFs to the lytic process. In the flagellum, the plasma membrane easily gives way, but the FS and the ODFs appear to be tough, and between the two, the FS appears to be tougher. Thus, this study incidentally reflects on the physical and biochemical nature of the various region-specific cellular organelles of the sperm with special reference to the response to the disintegration.
Koyanagi and Nishiyama[26] studied the disintegration of spermatozoa in the infundibular sperm-host glands of the fowl 15 days after artificial insemination. According to these authors, the plasma membrane of spermatozoa becomes obscure at an early stage of disintegration. The acrosome and nucleus of the head set out the disintegration process, and then the mitochondria of the middle piece and, finally, the principal piece-axial-filament complex and FS will undergo the disintegration. Although the specifics could be different, the sequence mentioned is in good agreement with our observation.
It is reported that the normal mammalian epididymis is an organ particularly rich in acid hydrolases such as β-galactosidase, N-acetyl-β-D-glucosaminidase, α-mannosidase, β-glucuronidase, aryl sulfatase, besides the DNases and other nucleolytic enzymes, consistent with a developed lysosomal apparatus for the disintegration of sperm proteins and DNA and are not functional to any substantial level just because of the predominance of the survival signals.[1,27] These enzymes may modify the spermatozoa as they pass through the epididymis or even disintegrate the spermatozoa.[28] Further, Jones[1] postulated that the perturbations that take place within the epididymal lumen following androgen depletion and collateral regression of the epithelium can activate death pathways that eventually lead to disintegration and dissolution of spermatozoa, as in the case of male animals with a seasonal reproductive cycle. The solubilization of several organelles, including the sperm head, mitochondria, FS, and ODFs, would be facilitated by the activation of these proteinases and hydrolases in the luminal fluid as a result of changes in pH and ionic strength due to the perturbations. For instance, during toxicity, like with that of AFB1 in the present study, the testosterone level is significantly lower[29,30] and might have contributed to the triggering of death signal and consequent elevated expression of these lytic enzymes.
During the epididymal sojourn, dead/defective spermatozoa pick up the epididymal sperm binding protein 1 (ELSPBP1) from the epididymosomes,[31,32] which contributes to the dense matrix in which the spermatozoa undergo disintegration.[18] This allows for the tagging of spermatozoa that must eventually be expelled while shielding the live spermatozoa from the detrimental consequences of the dead/defective spermatozoa.[32] Nonetheless, ELSPBP1 is not the sole protein that has been linked to defective spermatozoa. Among the many epididymosomal proteins known, programmed cell death 6, which is encoded by the apoptosis-linked gene 2, an apoptosis-related gene,[33] is implicated in the elimination of defective spermatozoa.[34] Annexin V, yet another protein found in the epididymosomes,[34] is involved in apoptosis[35] and is concerned with the removal of defective spermatozoa.[36] Therefore, epididymosomes being a part of the dense matrix where the spermatozoa undergo disintegration and liquefaction,[18] these epididymosomal proteins, which have an affinity to the defective spermatozoa might contribute to their disintegration and liquefaction.
In the epididymis, in addition to the DNase, other nucleolytic enzymes and the proteinases mentioned, ubiquitin and ubiquitin-C-terminal hydrolase, PGP9.5, are also expressed,[37] where ubiquitin appears to be secreted in the epididymal lumen.[38] Sutovsky et al.[39] were the first to introduce the idea of ubiquitination of sperm autoantigens concerning the phagocytosis of dead or defective spermatozoa by epididymal epithelium but, subsequently, extended it to include spermatozoal disintegration in the epididymal lumen.[40] The fidelity of the ubiquitin-proteasome system would be used to ensure that only defective spermatozoa are ubiquitinated and possibly disintegrated through ubiquitin conjugation, most likely through epididymosomes.[4] Altering a defective sperm’s surface could also stop the immune system from reacting to its defective parts, perhaps shielding the reproductive system against autoimmune infertility.[40,41] Dot blot analysis by Baska et al.[4] revealed that the caput fluid and caput spermatozoa had greater ubiquitin contents than the cauda fluid and cauda spermatozoa. When comparing the epithelia, the initial segment’s epithelium had the greatest ubiquitin content, followed by the caput and cauda epithelium.[4] This is in coherence with the appearance of the dense material, in which these defective spermatozoa undergo disintegration from the caput epididymis onward, which is again beefing up the view.
Endogenous proteasomes, acrosomal proteases such as acrosin and DNases, in addition to their counterparts in the epididymis, are also thought to contribute to the sperm disintegration process.[42,43] Furthermore, it is speculated that the epididymis can recognize spermatozoa with gross deformations as well as those with cryptic defects such as DNA fragmentation and that tagging a spermatozoon with ubiquitin could mitigate sperm numbers by directly activating apoptotic pathways within the sperm cells, making defective cells prone to phagocytosis or liquefaction in the epididymis, or both.[40] Therefore, the dense material ensuing from the epididymosomes could be used as a device to mass-ubiquitinate the defective spermatozoa while keeping viable spermatozoa away from the ubiquitination system. This raises the concern as to what would be the fate of the dense material once the spermatozoa have completely liquefied. Drawing inferences from what Martan[44,45] observed, it may be inferred that the dense masses containing the product of sperm disintegration and dissolution are sent out in the ejaculate or internalization, followed by recycling is another possibility.
CONCLUSION
Thus, this study suggests that the epididymis has a sperm quality control mechanism through the secretion of a dense material that segregates the defective/unviable spermatozoa for their complete disintegration and liquefaction involving roles for proteinases and nucleases. Further, the spermatozoa’s head, mid-piece, and principal piece follow different courses in the disintegration process.
Acknowledgments
We gratefully acknowledge the instrumentation facility provided by the DST under the FIST scheme (No. SR/FST/LSI-112/2002) and the grant provided by the University Grants Commission (UGC), Government of India, New Delhi, under the Special Assistance Programme (SAP) (No. F.3-5/2007 (SAP-II)) to the Department of Animal Science of our University. We thank the TEM facility at the Christian Medical College and Hospital (CMC&H), Vellore, India.
Ethical approval
The research/study was approved by the Animal Ethics Committee at Bharathidasan University, number 148, dated 06.04.2008.
Declaration of patient consent
Patient consent is not required as there are no patients in this study.
Conflicts of interest
There are no conflicts of interest.
Use of artificial intelligence (AI)-assisted technology for manuscript preparation
The authors confirm that there was no use of artificial intelligence (AI)-assisted technology for assisting in the writing or editing of the manuscript and no images were manipulated using AI.
Financial support and sponsorship
Prof. M.A. Akbarsha received funding for the work from the Department of Science and Technology (DST), Government of India, New Delhi (No. SR/SO/AS-59/2004).
References
- Sperm survival versus degradation in the Mammalian epididymis: A hypothesis. Biol Reprod. 2004;71:1405-11.
- [CrossRef] [PubMed] [Google Scholar]
- Sperm maturation in the domestic cat. Theriogenology. 2006;66:14-24.
- [CrossRef] [PubMed] [Google Scholar]
- A study of the function of the epididymis: IV. The fate of non-ejaculated spermatozoa in the genital tract of the male guinea-pig. J Exp Biol. 1931;8:163-75.
- [CrossRef] [Google Scholar]
- Mechanism of extracellular ubiquitination in the mammalian epididymis. J Cell Physiol. 2008;215:684-96.
- [CrossRef] [PubMed] [Google Scholar]
- Sperm biology and male reproductive health. Sci Rep. 2020;10:21879.
- [CrossRef] [PubMed] [Google Scholar]
- Role of posttranslational protein modifications in epididymal sperm maturation and extracellular quality control. Adv Exp Med Biol. 2014;759:159-80.
- [CrossRef] [PubMed] [Google Scholar]
- Clinical significance of the low normal sperm morphology value as proposed in the fifth edition of the WHO Laboratory Manual for the Examination and Processing of Human Semen. Asian J Androl. 2010;12:47-58.
- [CrossRef] [PubMed] [Google Scholar]
- An illustration of human sperm morphology and their functional ability among different group of subfertile males. Andrology. 2018;6:680-9.
- [CrossRef] [PubMed] [Google Scholar]
- Measurement and significance of sperm morphology. Asian J Androl. 2011;13:59-68.
- [CrossRef] [PubMed] [Google Scholar]
- The current perspective on genetic and epigenetic factors in sperm maturation in the epididymis. Andrologia. 2021;53:e13989.
- [CrossRef] [PubMed] [Google Scholar]
- Toxic effects and possible mechanisms of deoxynivalenol exposure on sperm and testicular damage in BALB/c mice. J Agric Food Chem. 2019;67:2289-95.
- [CrossRef] [PubMed] [Google Scholar]
- Observations on dag-like defect of spermatozoa induced by treatment of the phytotherapeutic Quassia amara/quassin in the mouse model. Andrologia. 2021;53:e14046.
- [CrossRef] [Google Scholar]
- Gonadal and epididymal sperm reserves in the rabbit: Estimation of the daily sperm production. J Reprod Fertil. 1968;15:15-25.
- [CrossRef] [PubMed] [Google Scholar]
- Sperm granuloma and reversibility of vasectomy. Lancet. 1977;2:588-9.
- [CrossRef] [PubMed] [Google Scholar]
- Degradation of spermatozoa in the epididymis of a seasonally breeding mammal, the rock hyrax, Procavia capensis. J Reprod Fertil. 1972;30:447-50.
- [CrossRef] [PubMed] [Google Scholar]
- Fine structural changes in the epididymal epithelium of moles (Talpa europaea) throughout the year. J Reprod Fertil. 1976;47:47-54.
- [CrossRef] [PubMed] [Google Scholar]
- A dense matrix of epididymal origin to process and remove defective spermatozoa: Observation in afb1-treated rat. J Endocrinol Reprod. 2008;12:53-56.
- [Google Scholar]
- Role of aposomes and epididymosomes in sperm quality control: A light and transmission electron microscopic study in an experimental rat model. Andrologia. 2021;53:e13862.
- [CrossRef] [Google Scholar]
- Quantitative and qualitative characteristics of the stages and transitions in the cycle of the rat seminiferous epithelium: Light microscopic observations of perfusion-fixed and plastic-embedded testes. Biol Reprod. 1990;43:525-42.
- [CrossRef] [PubMed] [Google Scholar]
- Pale vacuolated epithelial cells in epididymis of aflatoxin-treated mice. Reproduction. 2001;122:629-41.
- [CrossRef] [PubMed] [Google Scholar]
- Spermatotoxic effect of aflatoxin B(1) in the albino mouse. Food Chem Toxicol. 2003;41:119-30.
- [CrossRef] [PubMed] [Google Scholar]
- A formaldehyde-glutaraldehyde fixative of high osmolarity for use in electron microscopy. J Cell Biol. 1961;27:137A.
- [Google Scholar]
- Ultrastructure of epithelial cells in the epididymal region of the turkey (Meleagris gallopavo) J Anat. 1977;124:765-78.
- [Google Scholar]
- Spermatotoxic effect of aflatoxin B1 in rat: Extrusion of outer dense fibres and associated axonemal microtubule doublets of sperm flagellum. Reproduction. 2008;135:303-10.
- [CrossRef] [PubMed] [Google Scholar]
- The activity of some nucleolytic enzymes in semen and in the secretion of the male reproductive tract. Andrologia. 1977;9:15-22.
- [CrossRef] [PubMed] [Google Scholar]
- Disintegration of spermatozoa in the infundibular sperm-host glands of the fowl. Cell Tissue Res. 1981;214:81-7.
- [CrossRef] [PubMed] [Google Scholar]
- Compartmentalization of lysosomal enzymes in cauda epididymis of normal and castrated rats. Arch Androl. 2002;48:193-201.
- [CrossRef] [PubMed] [Google Scholar]
- N-acetyl-beta-D-hexosaminidase in the epididymis after vasectomy. Arch Androl. 1978;1:275-6.
- [CrossRef] [PubMed] [Google Scholar]
- Aflatoxin B1-induced reproductive toxicity in male rats: Possible mechanism of action. Int J Toxicol. 2014;33:155-61.
- [CrossRef] [PubMed] [Google Scholar]
- Effect of aflatoxins on testicular steroidogenesis and amelioration by vitamin E. Food Chem Toxicol. 2002;40:669-72.
- [CrossRef] [PubMed] [Google Scholar]
- Epididymosomes transfer epididymal sperm binding protein 1 (ELSPBP1) to dead spermatozoa during epididymal transit in bovine. Biol Reprod. 2012;87:94.
- [CrossRef] [PubMed] [Google Scholar]
- Epididymosomes: A heterogeneous population of microvesicles with multiple functions in sperm maturation and storage. Asian J Androl. 2015;17:726-9.
- [CrossRef] [PubMed] [Google Scholar]
- Interfering with apoptosis: Ca(2+)-binding protein ALG-2 and Alzheimer's disease gene ALG-3. Science. 1996;271:521-5.
- [CrossRef] [PubMed] [Google Scholar]
- Protein composition of human epididymosomes collected during surgical vasectomy reversal: A proteomic and genomic approach. Hum Reprod. 2008;23:1698-707.
- [CrossRef] [PubMed] [Google Scholar]
- Analysis of apoptosis by flow cytometry In: Flow cytometry protocols. Berlin: Springer; 1998. p. :217-38.
- [CrossRef] [PubMed] [Google Scholar]
- A novel approach for the selection of human sperm using annexin V-binding and flow cytometry. Fertil Steril. 2009;91:1285-92.
- [CrossRef] [PubMed] [Google Scholar]
- Activation of a UBC4-dependent pathway of ubiquitin conjugation during postnatal development of the rat testis. Dev Biol. 1999;212:217-28.
- [CrossRef] [PubMed] [Google Scholar]
- Light and electron microscopic immunohistochemical localization of protein gene product 9.5 and ubiquitin immunoreactivities in the human epididymis and vas deferens. Biol Reprod. 1996;55:291-7.
- [CrossRef] [PubMed] [Google Scholar]
- A putative, ubiquitin-dependent mechanism for the recognition and elimination of defective spermatozoa in the mammalian epididymis. J Cell Sci. 2001;114:1665-75.
- [CrossRef] [PubMed] [Google Scholar]
- Ubiquitin-dependent proteolysis in mammalian spermatogenesis, fertilization, and sperm quality control: Killing three birds with one stone. Microsc Res Tech. 2003;61:88-102.
- [CrossRef] [PubMed] [Google Scholar]
- Region-specific expression and secretion of the fibrinogen-related protein, fgl2, by epithelial cells of the hamster epididymis and its role in disposal of defective spermatozoa. J Biol Chem. 2004;279:51266-74.
- [CrossRef] [PubMed] [Google Scholar]
- Ability of hamster spermatozoa to digest their own DNA. Biol Reprod. 2003;69:2029-35.
- [CrossRef] [PubMed] [Google Scholar]
- Structural features of the 26S proteasome complex isolated from rat testis and sperm tail. Mol Reprod Dev. 2000;57:176-84.
- [CrossRef] [PubMed] [Google Scholar]
- Epididymal histochemistry and physiology. Biol Reprod. 1969;1:1134-54.
- [CrossRef] [PubMed] [Google Scholar]
- Occurrence of the intact spermatozoa in spontaneous ejaculations of isolated male guinea pigs. Trans Ill State Acad Sci. 1966;59:78-80.
- [Google Scholar]