Translate this page into:
Multiorgan damage and dysfunction due to infection by severe acute respiratory syndrome coronavirus-2 and post-recovery complications
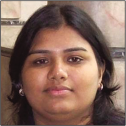
*Corresponding author: Vandana Chauhan, Centre for Medical Biotechnology, Amity Institute of Biotechnology, Amity University, Noida, Uttar Pradesh, India. chauhanvandana01@gmail.com
-
Received: ,
Accepted: ,
How to cite this article: Singh IR, Parayitam MR, Chauhan V, Vermani M, Yadav SS. Multiorgan damage and dysfunction due to infection by severe acute respiratory syndrome coronavirus-2 and post-recovery complications. J Reprod Healthc Med 2021;2:9.
Abstract
Large clusters of patients were reported with pneumonia such as symptoms of unknown causes, all linked to a seafood, and wet animal wholesale market in the city of Wuhan, Hubei Province, China in December 2019. This was followed by studies conducted by the Chinese authorities leading to the detection of a novel strain of coronavirus named as 2019-novel coronavirus. Further studies on the viral genome indicated that the virus is possibly of bat-origin belonging to the genus Betacoronavirus and family Coronaviridae. The name of the virus was changed to severe acute respiratory syndrome coronavirus-2 (SARS-CoV-2) due to its high similarity with SARS-CoV-1 and the disease was named coronavirus disease-2019 (COVID-19). The disease was declared a pandemic by the WHO by March 12, 2020. As of December 17, 2020, the total cases have been reported to be around 7.29 million with 1.63 million deaths worldwide. Symptoms of the disease can range from severe pneumonia requiring mechanical ventilation to basic symptoms such as fever and cough sometimes even being asymptomatic. Diagnostic tests include different laboratory based, point-of-care, and serological tests for controlling the spread of the disease. It has been indicated that the virus shows its effect by complete immune dysregulation due to excessive release of cytokines which is known as “cytokine storm” leading to the effect on multiple organs mainly the lungs through pneumonia such as symptoms, liver by causing severe damage indicated by high aspartate aminotransferase and alanine aminotransferase levels, kidneys through renal damage, and neurological symptoms being reported in some cases. It also affects circulatory systems through blood coagulation, skin damage, etc. Different symptoms have also been observed post recovery in the respiratory, cardiovascular, endocrine, and immune systems. Currently used treatment strategies are mostly symptomatic that includes use of invasive and non-invasive ventilation and broad spectrum antibiotics and steroids. Furthermore, different novel treatments are also being developed. It is extremely important to conduct further research on the effects of the disease on different organs and post-recovery symptoms to not only develop better diagnostic tools and treatment strategies but also to help people that have already recovered from the disease.
Keywords
Severe acute respiratory syndrome coronavirus-2
Coronavirus disease-2019
multiorgan dysfunction
Post-recovery
Pneumonia
hypoxia
Reverse transcription polymerase chain reaction
Treatment
INTRODUCTION
In early December 2019, large groups of patients were reported with symptoms like pneumonia of unknown causes, all linked to a seafood, and wet animal wholesale market in the city of Wuhan, Hubei province, China.[1] On December 31, 2019, after an alert being issued by the Wuhan municipal health commission, rapid response teams were sent from the China Center for Disease Control and Prevention (China CDC) with a notification sent to the WHO. They reported, through the bronchoalveolar lavage samples of the patients, the presence of a novel strain of coronavirus which was named 2019-novel coronavirus and then changed to severe acute respiratory syndrome coronavirus-2 (SARS-CoV-2).[2] The disease caused by the virus was named by the WHO as coronavirus disease-2019 (COVID-19). As December 17, 2020, 72,556,942 confirmed cases 1,637,155 deaths with 609,507 cases, and 13,458 deaths being reported in the past 24 h. It has affected 216 countries.[3]
The coronaviruses is a subfamily of RNA virus family – Coronaviridae, mostly found in bats. This virus has a positive sense single stranded RNA which is 26–32 kb in length and a nucleocapsid of helical symmetry.[4] The name “coronavirus” is derived from their outer fringe of embedded envelope glycoproteins creating an image reminiscent of the solar corona or halo, when observed through electron microscopy. These viruses are further classified into four genera named Alphacoronaviruses (alphaCoV), Betacoronaviruses (betaCoV), Gammacoronaviruses (GammaCoV), and deltacoronaviruses (DeltaCoV).[5] At least six different strains of coronaviruses have been identified that infect humans, all belonging to the Alphacoronavirus and Betacoronavirus strains which include:
-
Alphacoronaviruses
HCoV-229E
HCoV-NL63.
-
Betacoronaviruses.
HCoV-HKU1
SARS-CoV1
MERS-CoV
HCoV-OC43.
The SARS-CoV-2 coronavirus strain has been found to be a part of the Betacoronavirus genus (forming a clade within the sarbecovirus subgenus) where it has been found to show 79% and 51.8% identity with SARS-CoV 1 and MERS-CoV along with high similarity with a bat SARS like coronavirus RaTG13 (96% identity), respectively. SARS-CoV 2 is generally spherical with some pleomorphism and is about 60–120 nm in diameter. The virus consists of a nucleocapsid (N) protein which wraps the complete RNA genome consisting of a 5’ cap structure and 3’poly A tail. It also consists of a viral envelope (V) protein containing Matrix protein (M) and Spike Proteins (S).[1,5,6] The spike proteins consist of two major subunits S1 and S2 where S2 consists of a fusion protein, a transmembrane domain, and a cytoplasmic domain. Genetic analysis of samples from 103 patients indicates that there are two basic strains of the virus – L type and S type the former being more aggressive and infectious.[5]
It has been found that the virus has a high/strong binding affinity toward the human angiotensin converting enzyme 2 receptor (ACE-2) which is similar to the action of SARS-CoV-1 (~76% amino acid identity shared between them) also requiring the use of a cellular serine protease enzyme TMPRSS2 required for S1/S2 cleavage (S priming) which is essential for virus entry into the host cells.[7]
Apart from the differences in the genomes of MERS, SARSCoV1, and SARS-CoV-2, there are other differences existing among the viruses listed as under:
Phylogenetic analysis indicating that SARS CoV-2 and SARS CoV-1 are considered to be part of the same clade (clade I) and with MERS-CoV part of a different Clade (Clade II)
It has been speculated that SARS-CoV-2 has a higher binding efficiency in comparison with SARS-CoV-1
SARS-CoV-2 has the lowest case fatality compared to SARS-CoV-1 and MERS-CoV, 2.3%, 9.5%, and 34.4%, respectively[8]
SARS-CoV-2 and SARS-CoV-1 use the ACE2 receptor for entry while dipeptidyl peptidase 4 receptor is used by MERS- CoV as a means of cell infection
SARS-CoV-2 has the highest reproduction number (R0) value among the three viruses indicating a higher pandemic potential, with the speculated R0 values are 2–2.5, 1.7–1.9, and <1, respectively.[8]
Basic symptoms include cough, sputum production, shortness of breath, fever, sore throat, fatigue, and headache along with some uncommon symptoms such as vomiting and diarrhea. The basic incubation period of the virus was found to be around 1–14 days with median around 5.1 days. Based on the present data, it has been indicated that the pathogenic mechanism is rather complex involving both the innate and adaptive immune systems to work in a synergistic manner on infection leading eventually to immune dysregulation due to induction of a severe cytokine response, that is, cytokine storm, eventually leading to extensive tissue damage.[9]
With the primary goal of the pandemic is controlling the spread of the disease among people and since there is no proper cure for the disease and vaccines have not yet been distributed and administered, it is extremely important to reduce the number of susceptible persons which can be done through identification and isolation of the positive cases.[a] This can only be done with the help of different clinical diagnostic tests. These tests are an integral part of the different strategies that have been adopted by different countries for the containment of the disease.[10]
Out of the different tests that are currently used, the laboratory-based Reverse transcription polymerase chain reaction (RT-PCR) tests is considered to be the reference standard for the diagnosis of the disease. Nasopharyngeal swabs are collected from the patients, followed by RNA extraction and the quantitative detection using RT-PCR. This test has been essential for disease detection but there are major limitations such as long time duration, requirement of multiple tests for proper diagnosis, and occurrence of false negatives and false positive results associated with it.[10] Other testing tools have also been developed in the form of point-of-care tools that facilitate testing outside of laboratory settings through the detection of the viral proteins in respiratory samples but based on current evidence and WHO, their use is recommended only in research settings. Antigen based point of care tests is also being developed for rapid detection.[11]
Serological tests such as ELISA involving the detection of IgG and IgM antibodies have been developed that are accurate and easy to perform. However, they have a major limitation as they are effective only during the onset of infection when the risk for virus shedding and transmission is the highest. Studies have shown that they can be mainly used for epidemiological studies.[10]
Chest imaging techniques in the form of chest radiography, chest CT (computed tomography), and chest ultrasound have also been used for the diagnosis of the disease. According to the WHO, it is recommended to use these techniques only during situations where RT-PCR tests are difficult to perform and during the monitoring and treatment of patients having severe symptoms. It is not considered to be used in place of RT-PCR test, but it can be used in conjunction with it.[12] Chest radiography, although has a lower sensitivity than chest CT scans, it is much safer and can be conducted easily in mobile laboratories having portable equipment, chest CT scans are preferred in situations where patient has severe symptoms and is not responding to any supportive treatment.[11]
Even with the presence of different diagnostic tools that are currently used for the diagnosis of different symptomatic patients, there are some gaps present mainly in the screening of asymptomatic patients in the incubation phase of the disease, detection of virus shedding in patients in the convalescent phase and also in the development of cheaper tools that do not require well equipment laboratories for testing and curbing the spread in remote areas. Novel technologies in the form of CRISPR-based technologies are being used in the development of temperature stable, low cost, and rapid diagnostic tools that can be deployed in remote regions.[10]
ORGANS AFFECTED BY COVID-1
Lungs
It has been reported that all patients suffering from COVID-19 showed effects on the lungs ranging from mild-to-severe cases of pneumonia requiring the use of mechanical ventilation and intensive care to counter hypoxia.
Various imaging studies have been done on the lungs of different patients using CT scans. According to these studies, it has been found that patients show multifocal, patchy ground glass opacities (GGOs) with interlobular septal, and vascular thickening present mostly in the peripheral regions of the lungs.[13] The morphology of these GGOs is initially round and patchy, converting to linear and triangular ones similar in appearance to a “spider web.” Furthermore, “crazy paving” patterns can be observed along with cavitation, nodules, pleural effusion, and lymphadenopathy in rare cases.[13,14] The pathological indication of the GGOs is diffused alveolar damage and the histological indication is presence of blood, pus, water, or cells in alveoli.
Studies have also indicated that changes occur in the chest CT scans as the disease progresses. Scans of patients in early stages (0–2 days after occurrence of symptoms) show mild damage in the form of small, lobular and sub segmental, patchy GGOs with interstitial changes, and thickening of vascular lumens. Most patients also show normal CT scans. Patients in the peak and progressive stages show lesions along with multiple GGOs, dense consolidation in lobes (lung tissues that are filled with air instead are filled with liquid) along with “Crazy Paving” signs. Severe patients show diffuse lesions appearing as “white lungs” in both lungs.[15] These symptoms observed are found to be highly identical to the symptoms observed in other related diseases especially in the case of SARS-1.[13]
Liver
Studies conducted in the “Fifth medical Center of PLS general Hospital, Beijing, China” have shown that 2–11% patients of COVID-19 depicted some form of liver problems.[16] Approximately 14–53% cases reported an unusual amount of aspartate aminotransferase (AST) and alanine aminotransferase (ALT) during the progression of the disease within them.[16] In COVID-19 patients with severe liver dysfunction, AST was found to be 63%, eight out of 13 patients required intensive care with seven (25%) out of 28 patients not requiring any intensive care in ICU.[17] One interesting thing observed here was that AST levels in subclinical phase patients were found to be extremely low than AST of patients diagnosed.
Liver damage is more common in mild COVID-19 patients where liver cells might be damaged by direct viral infection. This point has been proven by detection of SARS-CoV 2 RNA in 2–10% of COVID-19 patients with diarrhea symptoms.[16]
Both SARS-CoV and SARS-CoV-2 have been found to infect liver cells due to the presence of the ACE2 receptor.[18] It was also noted at time of SARS-1 that many patients had virus or presence of virus material in liver tissue, but viral inclusion was not observed. Furthermore, MERS viral patients did not have any detectable presence of virus in the liver. In the work of Zhang et al. at the Fifth Medical Center of PLA General Hospital, National Clinical Research Center for Infectious Diseases, Beijing, 30 of 56 patients with COVID-19 were found to show presence of GGT biomarker, specific for detection of cholangiocyte injury.[16] They also found 1 of 56 patients had elevated level of alkaline phosphatase leading to the conclusion that ACE2 receptor has higher level in cholangiocytes, indicating that virus might directly infect to the liver and cause injury.[16] However, analysis of liver tissue from deceased patients did not indicate any viral inclusions in liver which led to the proposition of another theory that liver damage is a consequence of drug hepatotoxicity rather that direct viral infection. Furthermore, immune-mediated inflammation and pneumonia with hypoxia also contribute or increase chances of liver injury in case of critical COVID-19 patients. Mild (less severe) patients of COVID-19 often recover from liver damage without any special treatment.
Even though chronic liver disease is more common in highly populated countries, studies must be conducted describing the relationship between liver injury due to COVID-19 and different existing liver diseases. Furthermore, due to the heavy burden and their overall immunocompromised status, patients with any previous history of liver ailments have more chances to be affected by COVID-19.[16]
Kidney
Acute kidney injury (AKI) was reported more frequently than extra pulmonary complication (29%), compared to liver (23%), and cardiac (23%) ailments in 52 critically patients reported for ICUs.[19] Furthermore, 25% of AKI patients needed regular renal replacement therapy, 80% deaths with a median duration was seen for 7 days from ICU admission to death[19] SARS-CoV-2 can also be detected in urine sample of patients and indicate urinary tract infection.[20]
Studies conducted on COVID-19 patients have shown high renal dysfunction indicated by acute tubular necrosis along with degradation of vacuoles and luminal brush border sloughing in different areas. It has also been shown that SARS-CoV-2 antigen was found accumulated in renal epithelial tubules, indicating SARS-CoV-2 may directly infect the human kidney organs, leading to kidney dysfunction.[20] The direct infection of kidney can also be supported by presence of ACE2 receptor in kidney cells, that is, mainly the podocyte cells and proximal tubule cells[21] which is the target receptor for SARS-COV-2. Furthermore, since ACE2 binding of SARS-CoV-2 is greater than SARS-CoV, it allowed incorporation of larger viral load in cells of many organs, especially in the kidney, which again act as viral reservoirs.[22]
Plasma protein fractions are filtered at renal glomeruli and most of them are reabsorbed in the proximal tubule; hence, negligible amount of protein is retained in healthy urine. The filtration barrier is dependent on the three main components, endothelial cell, glomerular basement membrane, and podocytes.[18,22] The podocyte cells are sensitive to renin angiotensin aldosterone system homeostasis, due to specific expression of ACE2 in this region.[19] If ACE2 expression is abnormal, it can lead to dysfunction of podocytes and this sheer stress may result in single nephron hyperfiltration eventually leading to AKI. The AKI was found mostly in critically ill patients.[23] These patients in ICU had higher level of TNF-a, IL-1b, IFN-g, and IL-8 compared to non-critically ill patients[24,25] which is indicative of the “cytokine storm” where the syndrome can cause inflammatory response and lead to kidney dysfunction. At least 20% of the infected patients reported occurrence of hematuria caused by kidney damage and extra-renal abnormalities in a study.[26]
Cardiovascular system
Studies have indicated that patients suffering with COVID-19 have shown different cardiovascular symptoms, mainly in serious patients. Immune system disruption along with increased metabolism and higher coagulant activity result in increased risk to COVID-19 related cardiovascular disease (CVD). Patients suffering from different comorbidities, especially those of cardiovascular nature have shown a higher risk and severity of COVID-19 infection as indicated by a study done on 44,672 patients showing a 5 time increase in mortality by COVID-19 in patients with CVD history. At least 20% of all patients displayed symptoms which include cardiovascular symptoms, the most severe being cardiogenic shock.[27,28] The main symptoms shown are:
Myocardial injury and myocarditis – These were found to be common in patients of earlier similar virus outbreaks-SARS-1 and MERS. This symptom was detected by higher than normal levels of biomarkers mainly Troponin 1 observed in 7–17% of non-severe patients and 22–31% of critical patients requiring intensive care. It has been a challenge in diagnosis of this symptom due to variety of clinical severity. The appearance in ECG analysis was found to sometimes mimic the appearance of acute coronary syndrome. Both ECG and echocardiographic differences observed have been found to act as an effective indicator toward the severity of the disease[28,29]
Acute myocardial infarctions – patients showed a higher risk to this symptom due to inflammation and hypercoagulation
Acute cardiomyopathy and heart failure – This was found as the primary manifestation in COVID-19 patients. Study conducted showed 33% of the patients with cardiac myopathy and 24% patients suffering heart failure with a higher mortality risk
Dysrhythmias – They have been observed in various forms in 7% of patients with sinus tachycardia being most common due to simultaneous, multiple causes such as fever, hypoxia, and anxiety.
Although investigation on exact mechanisms is undergoing at present, the potential mechanism considered is direct myocardial cell injury mainly of the internal cells containing the ACE2 receptor based on observation of patients of previous outbreaks, as detected in 35% of autopsied hearts of SARS patients.[29]
Nervous system
Mao et al., 2019, reported that approximately 36.4% of 214 patients of SARS-CoV-2 had neurological manifestations especially seen in patients with severe symptoms along with other health ailments such as hypertension.[30] These manifestations included cerebrovascular disease, impaired consciousness, ischemic strokes, cerebral hemorrhage, damage to skeletal muscles, and seizures. The manifestation of most symptoms was in the early periods of the disease. The cases were divided into three broad categories – central nervous system (CNS), cranial and peripheral nervous system manifestations, and skeletal muscle injuries. Two-thirds of the patients were agitated when sedation and neuromuscular blockade were withdrawn and 14% of patients were found to have acute respiratory distress syndrome (ARDS) symptoms.[30] About 5% had symptoms and were diagnosed with ischemic stroke and 1% with cerebral hemorrhage, their inflammatory response was monitored, and increased CRP and D-Dimer level were observed. Majority of population were old and several had hypertension and diabetes mellitus.[30]
Studies done in the Netherlands and France have shown that blood coagulation in body was at 20–30% in critically ill COVID-19 patients.[31] MERS-CoV, SARS-CoV had history of neuro invasion, also since they also bound to ACE2 receptor and cases of direct viral infection by COVID-19 in CNS was also noted, it was not a surprising thing that SARS-CoV-2 RNA was detected in CSF proven through genome sequencing but they were not detected in nasopharyngeal swabs.[32] It was also hypothesized that ACE2 receptor and cases of direct infection of CNS may also contribute to death of infected patients.[32,33] Direct infection might also be possible due to Vagus nerve presence.[33]
Due to malfunctioning of the respiratory system, studies have also hypothesized damage to the nervous system due to hypoxia and actions of the immune system, through development of severe inflammatory response syndrome.
Europe and Israel reported olfactory dysfunction around 30– 60 % of patients.[34,35] Furthermore, in 12 European Hospital around 85.6% showed olfactory dysfunction and 88% showed gustatory dysfunction.[36] In Italy, the number was around 65% and 11% that showed it before other symptoms and it was found to be more common in women. The recovery rate of olfactory and gustatory was around 25.5% over a 2-week period with some patients only recovering one of the senses.[37]
Other symptoms of COVID-19
Blood coagulation
Most of the serious COVID-19 patients admitted in hospitals have shown significant abnormal coagulation of blood showing high mortality.[38] A study was conducted in a hospital in Wuhan by Han et al. on 134 subjects (of which 94 were patients and 40 were normal) on different parameters that included antithrombin (AT), fibrin/ fibrinogen degradation products (FDP), prothrombin time (PT), fibrinogen (FIB), international normalized ratio (INR), activated partial thromboplastin time (APTT), prothrombin time activity (PT-act), and thrombin time (TT).[38] The results of the study showed lower levels of AT and PT-act in patients compared to the control group; consisting of all normal people, with no difference among the mild and severe patients and a significant difference between mild and severe patients, respectively.[38] Higher D-dimer levels along with FIB and FDP levels were also observed in the patients compared to the control group with a significant difference between the mild and critical patients in the D-dimer levels and no difference in FIB levels. No changes were observed in APTT, PT, PT-INR, and TT values compared to control.[38]
Another study conducted by Tang et al. has also shown that the major diagnostic parameter for disseminated intravascular coagulation (DIC) showed that 71.4% of cases (non-survivors) showed higher DIC especially in later stages of the disease with 4 days as the median time duration from admission to DIC.[39] Pulmonary embolisms along with venous thromboembolic events have also been observed in patients.[40]
The mechanism for abnormal coagulation, that is, DIC is due to sepsis with the development of DIC symptoms due to the activation and release of cytokines by monocytes and endothelial cells along with the presence of tissue factor, von Willebrand factor and circulation of free thrombin without any presence of anticoagulants leading to fibrinolysis. The higher levels of D-dimer and FDP are also suggested by studies to be used as an indicator for disease progression.[39]
Cutaneous manifestations
Although being rare compared to other symptoms, cutaneous symptoms have also been observed in COVID-19 patients. The most common manifestation has been morbilliform (measles like rash) shown by 36.1% of all patients along with other symptoms like:
Papulovesicular rash (34.7%)[41]
Urticaria (9.7%)[41]
Painful acral red purple papules (15.3%)[41]
Livedo reticularis lesions (2.8%)[41]
Petechiae (1.4%).[41]
Furthermore, about 19.4% of all patients have shown cutaneous lesions on hands and feet (soles extremities, etc.).[42,43] About 12.5% of all patients showed cutaneous symptoms before the onset of respiratory symptoms. All of the lesions healed within a period of 10 days in all patients.[38]
The underlying mechanism for all the cutaneous symptoms is not yet well known. Studies and research have postulated that the presence of virus particles in blood vessels of cutaneous tissue could lead to lymphatic vasculitis like those observed in the case of thrombophilic arteries due to activation of complexes in blood releasing cytokines.[41] It is also hypothesized that activation of Langerhans cells occurs after immune activation and livedo reticularis lesions occur due to microthrombosis that occur in other organs reducing blood flow to the cutaneous vascular system. Hypoxia related accumulation of deoxygenated blood and DIC can also further explain these complications.[41]
Effects on reproductive health
It is clearly known that the proper functioning of the human reproductive system has significant dependence on different physical, psychological, and social factors; the advent of the SARS-CoV 2 pandemic status along with all the medicines, treatment, and safety and control measures taken could have a potentially significant effect on the reproductive health of individuals.
-
Effect on the male reproductive system – Studies conducted by conducted by Wang et al. have shown the presence of high expression of the ACE2 receptors and the TMPRSS2 proteins in the testis on spermatogonia, Leydig and Sertoli cells, spermatids along with the seminiferous ducts.[44] Viral orchitis was reported to be one of the complications in patients infected by SARS CoV-1 and had shown importance of further studies on the effect reproductive health in recovered patients. A research study research had not only reported the presence of virus RNA in the semen of recovered patients but also indicated the presence of the virus in the seminal fluid of patients with acute infection[44]
It is also hypothesized that the male reproduction system is affected as it is known that the virus could cause dysfunction of autophagy mechanisms leading to damage of cells.[45] This indicates that more importance is given on studying the effects of the virus on reproductive health.
According to a study by Lee et al., 2020, people suffering with prostate cancer (without any treatment in the form of androgen deprivation therapy; odds ratio = 1.09 and p value of 0.82) have a higher risk for infection and death by COVID-19 due to the use of TMPRSS2 for internalization whose expression depends on androgen receptors.[46]
The different drugs used for the treatment of the virus have also been found to affect the male reproductive system. Antiviral drugs like ribavirin (in the presence of interferon) have been shown to affect the fertility in males through impaired spermatogenesis resulting in low sperm count as shown in clinical studies even after the termination of provision of the drug.[44] Glucocorticoids have been found to cause breakage of cell-cell connections, affect the blood-testis barrier, and cause germ cell apoptosis.[44]
-
Effect on the female reproductive system-ACE-2 receptor is found in ovary, uterus vagina and placenta and it plays an important role in balancing AngII and Ang(1-7) levels that play a role in regulation of follicle development, oocyte maturation influence on ovulation and other various stages of menstrual cycle.[47] The mRNA of ACE2 has also been identified in uterus and is more common in epithelial cells than in stromal cells which indicate that sexual transmission of virus particles might be possible but has to be further investigated in detail. AngII also functions in vascular bed and endometrium regeneration and is responsible for initiation of menstruation.[47] These pathways can be hindered if infection is found. Endometrium Ang II is important for regular menstrual cycles and its alteration can relate to dysfunctional uterine bleeding
Compared to male genital cancers, cancers of the female genital and breast cancer have been found to be at lower risk of infection and death from COVID-19 (female genital organs – odds ratio of 0.79, P = 0.70; breast cancer – odds ratio – 0.97 P = 0.70).[46]
Pregnant women are thought to be at a higher risk from specific respiratory morbidities such as H1N1 and pneumonia, as there are some similarities with COVID-19, they are thought to be at a significant risk than general population but current data does not support this fact.[48] Studies have shown that intense inflammatory response which has been reported in large number of cases is not seen in pregnancy due to relative immunosuppression which may be considered as a reason as to why they do not develop severe respiratory symptoms.[48] Transmission of virus through vertical transmission has been reported but more data are still needed for a proper conclusion. Cesarean section rates have been alternative from it and negligible transmission is seen in it.[48] In case of breastfeeding, virus was not found in milk samples but some level of risk of transmission is existent due to close contact with the mother.[48]
Although there is strong need on further experiments on the reproductive system to study effects of SARS-CoV-2, an experiment conducted on risk assessment of SARS-CoV-2 infection in the endometrium across the menstrual cycle done by Henarejos-Castillo et al. indicated that the endometrium lining in older women especially going through assisted reproductive treatments are found to be at higher risk of infection.[49]
COMPLICATIONS IN COVID-19 PATIENTS POST RECOVERY
Even though the disease has been extremely dangerous with a large number of casualties and a mortality rate of 3–5%, a high recovery rate has also been observed in most of the countries effected. At present, very less number of studies has been conducted in recovered patients that shed some light on the post recovery complications of COVID-19. The reason for the same might be due to anxiety of contracting the infection and with a highly variable time period for the occurrence of complications ranging from few weeks to decades after recovery. Research has indicated the presence of the SARS-CoV-2 virus in the blood of patients after recovery. Thus, to reduce the risk of further infection, to understand the disease in more detail and to determine and provide any form of post-acute care to recover from further infections or multiorgan damages.[44]
Studies performed on recovered patients have shown a clear decrease in the overall health post recovery.[44] Gastrointestinal tract, kidney, heart, brain, eyes, and lungs of patient may persist infection. A long-term relevance of is speculated to remain in them causing several problems such as AKI, ARDS, and weakened immune system. Presence of pulmonary fibrosis in lungs has been reported in studies conducted on SARS-1 and MERS patients indicating its possible occurrence in recovered COVID-19 patients thus requiring more stringent monitoring.
The “Cytokine storm” may cause inflammation problems where these inflammatory cytokines can induce demyelination or cell death in nervous system as indicated by studies that can lead to potential functional loss if cell death is not repaired after the recovery of patient from COVID-19. If CRS is treated with T-cell engaging therapies after COVID-19 recovery, sometimes it can lead to neurotoxicity, hallucinations, mild headache aphasia, hemiparesis, cranial nerve palsies, seizures, and somnolence. Stress, depression, fear, post-traumatic stress disorder, post-intensive care syndrome, obsessive compulsive disorder, and other psychological disorders have shown to occur in a study done of recovered SARS-1 patients.[45,48] Research based on the previous infections by other strains of HCoVs have indicated occurrence of psychotic disorders (schizophrenia, etc.) in patients indicating a possible relationship between psychotic disorders and SARS-CoV-2.
Studies have indicated a high risk to the cardiac system in recovered patients which are mainly due to the exposure of medicines used for treatment. Chloroquine and hydroxychloroquine anti-malarial drugs have been found to show some effect against the cause a lowering in pH leading to electrolyte imbalance along with direct myocardial toxicity, AV blocks, and longer QT intervals. Another antiviral medicine – remdesivir which has shown positive clinical trial results has also shown to cause arrhythmias and hypertension. Azithromycin has been shown to interact and inhibits antiarrhythmics, anticoagulants, statins, etc.[28]
Recovered patients have also shown to suffer from paranoia, stigmatization, and ostracized by members of the society due to the anxiety of catching of the infection which increases risk of depression, mental stress, etc.[44]
TREATMENT STRATEGIES FOR COVID-19
COVID-19 is rapidly spreading among different populations and unfortunately the different drugs that have been identified are still not approved for use. At present, due to the sudden onset of the disease, most hospitals are undergoing symptomatic treatment strategies. For severe cases of pneumonia, septic shock, and COVID-19 ARDS, continuous monitoring of patient vital signs is done in ICUs along with the use of non-invasive or invasive ventilation to counter the lack of blood oxygen saturation and different broad-spectrum antibiotics and glucocorticoids. Convalescent plasma therapy which involves the use of plasma of compatible patients is also being used in treatment as it contains antibodies against the disease but it is recommended for use only on patients with severe life -threatening symptoms.[12]
Along with symptomatic treatment, different treatment strategies are under development for the disease. The different treatment strategies for COVID-19 can be of two major types – drug-based therapy and immunotherapy.
The drug-based treatment involves the use of drugs that act by targeting different components of the virus life cycle. They are mainly of two major forms – viral replication inhibitors and cell entry blockers.[12] Cell entry blockers include chloroquine and hydroxychloroquine along with other drugs such as arbidol and camostat mesylate that act as ACE2 blockers or TMPRSS2 serine protease blockers that prevent virus entry. Viral replication inhibitors include an array of drugs that are antivirals blocking virus replication (with the help of nucleoside analogues such as remdesivir and favipiravir), antimalarials (i.e., Chloroquine and hydroxychloroquine) that alter lysosomal and endosomal pH thus preventing injection of viral genetic material and also blocking RNA polymerase activity[50] and many more. They are mostly used in conjunction and they are still in the clinical trial phase.
Immunotherapeutic approaches involve the activation of the body’s own innate immune system which include Type I interferons (mainly INF-Alpha), viral recognition through pattern recognition receptor, antigen presentation (T- Cells), and aberrant activation of myeloid cells (mainly macrophages). The immunotherapeutic approach involves mainly two treatment methodologies which are either used individually or in combination – expansion of effector T-cells (adaptive T-cell Therapy) or by blocking inflammatory cytokines while enhancing the NK cell activity and Type I interferons. Various novel strategies have also been developed and are under trials that involve the use of interleukins like IL-2 OR IL-7 in therapy, recombinant treatment which works by reversing lymphopenia and restoring T-cell count, constraining excessive inflammation and the use of monoclonal antibodies such as tocilizumab and sarilumab that are used in conjunction with antivirals.[12,51]
Along with the development of different drugs, different vaccines have also been developed with some candidates already under Phase 2 or 3 trials. COVAXIN developed by BHARAT biotech and COVISHIELD developed by the Serum Institute of India and University of Oxford are two of the important vaccine candidates that are approved for Phase 3 trials in India.[12]
CONCLUSION
Since the detection and outbreak of the disease in December of 2019, COVID-19 has completely stopped the world and affected millions of people all around the globe resulting in many deaths till date. Based on the findings, the present review illustrates that SARS-CoV-2 through binding with the ACE 2 receptor and the subsequent uncontrolled response of the immune system is resulting in a “cytokine storm” affecting different organs such as the lungs, kidneys, and liver along with other neurological and cardiovascular complications leading to death of the patients in many cases.
It is extremely important to conduct further research on understanding the contagious nature of the virus and the effects it has on the various organs of the body which, in turn, would help in proper understanding of the disease and lead to formation of better diagnosis tests, treatment protocols and precautionary measures that would help in further reducing the increase in the number of cases and reduce deaths. Emphasis also must be given on research conducted on the different post-recovery symptoms of the disease for understanding it even further and providing proper treatment on post-recovery complications arising in recovered patients.
Acknowledgment
We express our gratitude to Amity Institute of Biotechnology, Amity University Noida, Uttar Pradesh, for continuous support and motivation.
Declaration of patient consent
Patient’s consent not required as there are no patients in this study.
Financial support and sponsorship
Nil.
Conflicts of interest
There are no conflicts of interest.
References
- Features, evaluation and treatment Coronavirus (COVID-19) In: Stat Pearls. Treasure Island, FL: Stat Pearls; 2020.
- [Google Scholar]
- The SARS-CoV-2 outbreak: What we know. Int J Infect Dis. 2020;94:44-8.
- [CrossRef] [PubMed] [Google Scholar]
- Epidemiology, virology, and clinical features of severe acute respiratory syndrome-coronavirus-2 (SARS-CoV-2; Coronavirus Disease-19) Clin Exp Pediatr. 2020;63:119-24.
- [CrossRef] [PubMed] [Google Scholar]
- Full-genome sequences of the first two SARS-CoV-2 viruses from India. Indian J Med Res. 2020;151:200-9.
- [Google Scholar]
- COVID-19, immune system response, hyperinflammation and repurposing antirheumatic drugs. Turk J Med Sci. 2020;50:620-32.
- [CrossRef] [PubMed] [Google Scholar]
- The origin, transmission, and clinical therapies on Coronavirus disease 2019 (COVID-19) outbreak-an update on the status. Mil Med Res. 2020;7:11.
- [CrossRef] [PubMed] [Google Scholar]
- SARS-CoV-2 cell entry depends on ACE2 and TMPRSS2 and is blocked by a clinically proven protease inhibitor. Cell. 2020;181:271-80.e8.
- [CrossRef] [PubMed] [Google Scholar]
- COVID-19, SARS and MERS: Are they closely related? Clin Microbiol Infect. 2020;26:729-34.
- [CrossRef] [PubMed] [Google Scholar]
- Clinical course and outcomes of critically ill patients with SARS-CoV-2 pneumonia in Wuhan, China: A single-centered, retrospective, observational study. Lancet Respir Med. 2020;8:475-81.
- [CrossRef] [Google Scholar]
- Diagnostic testing for severe acute respiratory syndrome-related Coronavirus 2: A narrative review. Ann Intern Med. 2020;172:726-34.
- [CrossRef] [PubMed] [Google Scholar]
- Use of Chest Imaging in COVID-19: A Rapid Advice Guide, Ch. 3 Geneva: World Health Organization; 2020. p. :12.
- [Google Scholar]
- Diagnostic and treatment strategies for COVID-19. AAPS Pharm Sci Tech. 2020;21:222.
- [CrossRef] [PubMed] [Google Scholar]
- Chest CT features and their role in COVID-19. Radiol Infect Dis. 2020;7:51-4.
- [CrossRef] [PubMed] [Google Scholar]
- Imaging and clinical features of patients with 2019 novel Coronavirus SARS-CoV-2. Eur J Nucl Med Mol Imaging. 2020;47:1275-80.
- [CrossRef] [Google Scholar]
- A novel Coronavirus from patients with Pneumonia in China, 2019. N Engl J Med. 2020;382:727-33.
- [CrossRef] [PubMed] [Google Scholar]
- Liver injury in COVID-19: Management and challenges. Lancet Gastroenterol Hepatol. 2020;5:428-30.
- [CrossRef] [Google Scholar]
- Clinical features of patients infected with 2019 novel Coronavirus in Wuhan, China. Lancet. 2020;395:497-506.
- [CrossRef] [Google Scholar]
- Angiotensin-converting enzyme 2: SARSCoV-2 receptor and regulator of the renin-angiotensin system: Celebrating the 20th anniversary of the discovery of ACE2. Circ Res. 2020;126:1456-74.
- [CrossRef] [PubMed] [Google Scholar]
- Is the kidney a target of SARS-CoV-2? Am J Physiol Renal Physiol. 2020;318:F1454-62.
- [CrossRef] [PubMed] [Google Scholar]
- SARS-CoV-2 can be detected in urine, blood, anal swabs, and oropharyngeal swabs specimens. J Med Virol. 2020;92:1676-80.
- [CrossRef] [PubMed] [Google Scholar]
- Human Kidney is a Target for Novel Severe acute Respiratory Syndrome Coronavirus 2 (SARS-CoV-2) Infection, medRxiv No. 2; 2020
- [CrossRef] [Google Scholar]
- Identification of a potential mechanism of acute kidney injury during the COVID-19 outbreak: A study based on single-cell transcriptome analysis. Intensive Care Med. 2020;46:1114-6.
- [CrossRef] [PubMed] [Google Scholar]
- Renal histopathological analysis of 26 postmortem findings of patients with COVID-19 in China. Kidney Int. 2020;98:219-27.
- [CrossRef] [PubMed] [Google Scholar]
- Characterization of renin-angiotensin system enzyme activities in cultured SARS-CoV-2 affects kidney cells 31 mouse podocytes. Am J Physiol Renal Physiol. 2007;293:398-407.
- [CrossRef] [PubMed] [Google Scholar]
- Coronavirus disease 19 infection does not result in acute kidney injury: An analysis of 116 hospitalized patients from Wuhan, China. Am J Nephrol. 2020;51:343-8.
- [CrossRef] [PubMed] [Google Scholar]
- The approach to the patient with hematuria. Emerg Med Clin North Am. 2019;37:755-69.
- [CrossRef] [PubMed] [Google Scholar]
- Cardiac manifestations of Coronavirus In: Stat Pearls. Treasure Island, FL: Stat Pearls Publishing; 2020.
- [Google Scholar]
- Cardiovascular complications in COVID-19. Am J Emerg Med. 2020;38:1504-7.
- [CrossRef] [PubMed] [Google Scholar]
- COVID-19 and Cardiovascular disease. Circulation. 2020;141:1648-55.
- [CrossRef] [PubMed] [Google Scholar]
- Neurologic manifestations of hospitalized patients with Coronavirus disease 2019 in Wuhan, China. JAMA Neurol. 2020;77:683-90.
- [CrossRef] [PubMed] [Google Scholar]
- Neurological manifestations and neuroinvasive mechanisms of the severe acute respiratory syndrome Coronavirus Type 2. Eur J Neurol. 2020;27:1578-87.
- [CrossRef] [PubMed] [Google Scholar]
- The neuroinvasive potential of SARS-CoV2 may play a role in the respiratory failure of COVID-19 patients. J Med Virol. 2020;92:552-5.
- [CrossRef] [PubMed] [Google Scholar]
- Angiotensin-converting enzyme 2 in the brain: Properties and future directions. J Neurochem. 2008;107:1482-94.
- [CrossRef] [PubMed] [Google Scholar]
- Loss of Smell and Taste in Combination with Other Symptoms is a Strong Predictor of COVID-19 Infection. medRxiv 2020
- [CrossRef] [Google Scholar]
- Anosmia and Dysgeusia in Patients with Mild SARS-CoV-2 Infection. medRxiv 2020
- [CrossRef] [Google Scholar]
- Olfactory and gustatory dysfunctions as a clinical presentation of mild-to-moderate forms of the Coronavirus disease (COVID-19): A multicenter European study. Eur Arch Otorhinolaryngol. 2020;277:2251-61.
- [CrossRef] [PubMed] [Google Scholar]
- Alterations in smell or taste in mildly symptomatic outpatients with SARS-CoV-2 infection. JAMA. 2020;323:2089-90.
- [CrossRef] [PubMed] [Google Scholar]
- Prominent changes in blood coagulation of patients with SARS-CoV-2 infection. Clin Chem Lab Med. 2020;58:1116-20.
- [CrossRef] [PubMed] [Google Scholar]
- Abnormal coagulation parameters are associated with poor prognosis in patients with novel Coronavirus pneumonia. J Thromb Haemost. 2020;18:844-7.
- [CrossRef] [PubMed] [Google Scholar]
- Pulmonary embolism in patients with COVID-19: Time to change the paradigm of computed tomography. Thromb Res. 2020;190:58-9.
- [CrossRef] [PubMed] [Google Scholar]
- Cutaneous manifestations of COVID-19: Report of three cases and a review of literature. J Dermatol Sci. 2020;98:75-81.
- [CrossRef] [PubMed] [Google Scholar]
- Chilblain-like lesions on feet and hands during the COVID-19 pandemic. Int J Dermatol. 2020;59:739-43.
- [CrossRef] [PubMed] [Google Scholar]
- Follow-up studies in COVID-19 recovered patients-is it mandatory? Sci Total Environ. 2020;729:139021.
- [CrossRef] [PubMed] [Google Scholar]
- Potential risks of SARS-CoV-2 infection on reproductive health. Reprod Biomed Online. 2020;41:89-95.
- [CrossRef] [PubMed] [Google Scholar]
- The hypothesis that SARS-CoV-2 affects male reproductive ability by regulating autophagy. Med Hypotheses. 2020;143:110083.
- [CrossRef] [PubMed] [Google Scholar]
- COVID-19 prevalence and mortality in patients with cancer and the effect of primary tumour subtype and patient demographics: A prospective cohort study. Lancet Oncol. 2020;21:1309-16.
- [CrossRef] [Google Scholar]
- Potential influence of COVID-19/ACE2 on the female reproductive system. Mol Hum Reprod. 2020;26:367-73.
- [CrossRef] [PubMed] [Google Scholar]
- Clinical update on COVID-19 in pregnancy: A review article. J Obstet Gynaecol Res. 2020;46:1235-45.
- [CrossRef] [PubMed] [Google Scholar]
- SARS-CoV-2 infection risk assessment in the endometrium: Viral infection-related gene expression across the menstrual cycle. Fertil Steril. 2020;114:223-32.
- [CrossRef] [PubMed] [Google Scholar]
- COVID-19 treatment update: Follow the scientific evidence. J Thromb Thrombolysis. 2020;50:43-53.
- [CrossRef] [PubMed] [Google Scholar]
- Cell-mediated immunity to SARS-CoV-2. Pediatr Investig. 2020;4:281-91.
- [CrossRef] [PubMed] [Google Scholar]